Post by Dr. Sydney Boutros, Ph.D., recent graduate of Behavioral Neuroscience at OHSU.
NogginFest was an extraordinary community gathering this spring in Portland, Oregon – the largest free, all ages public celebration of live music, are brains and neuroscience research in the Pacific Northwest!
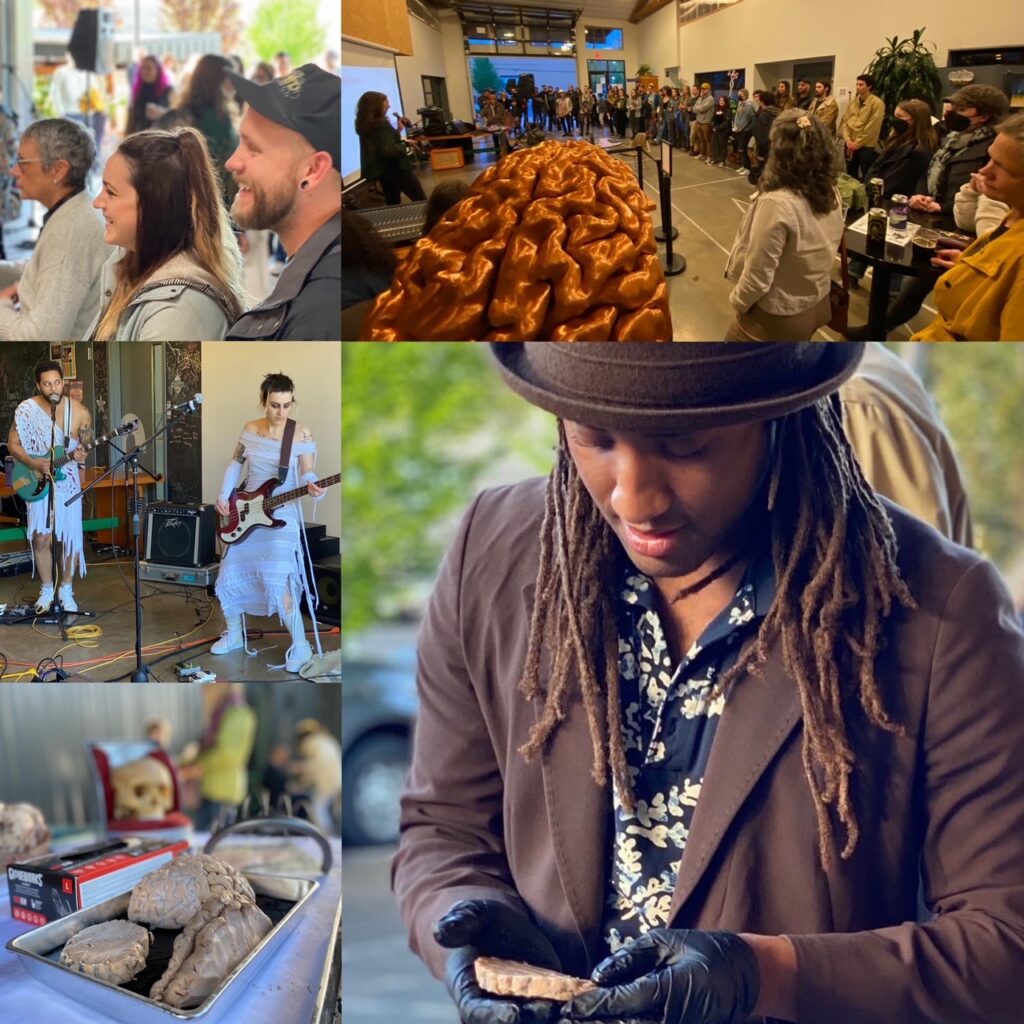
LEARN MORE: NogginFest 2022: A Mid-Pandemic Revival!
LEARN MORE: NOGGINFEST!
We were honored to hear from Sydney Boutros, who at this year’s NogginFest was a PhD candidate in Dr. Jacob Raber’s lab at OHSU. She is now Dr. Boutros, PhD! Sydney has collaborated with Noggin before, discussing her research at Floyd’s Coffee along with artist Kit S. Carlton.
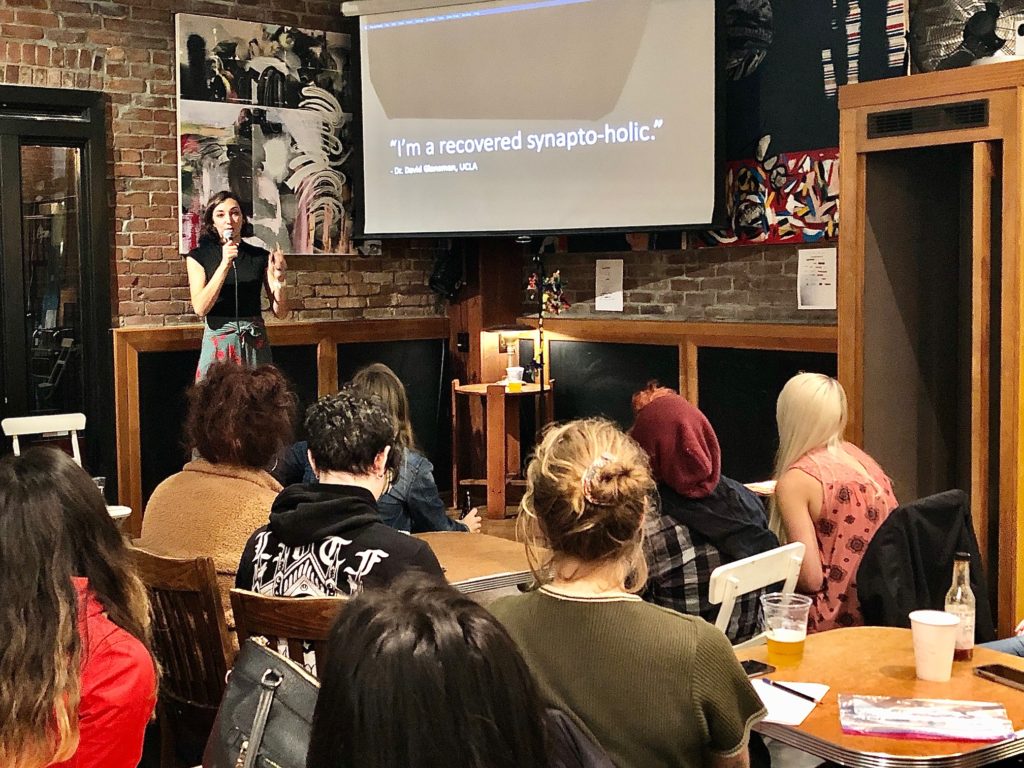
LEARN MORE: Memory, Poetry, Brains
From Dr. Sydney Boutros
Memories make us us. At NogginFest this year, I had the wonderful opportunity to share what I’ve learned about how memories come to form in our brains.
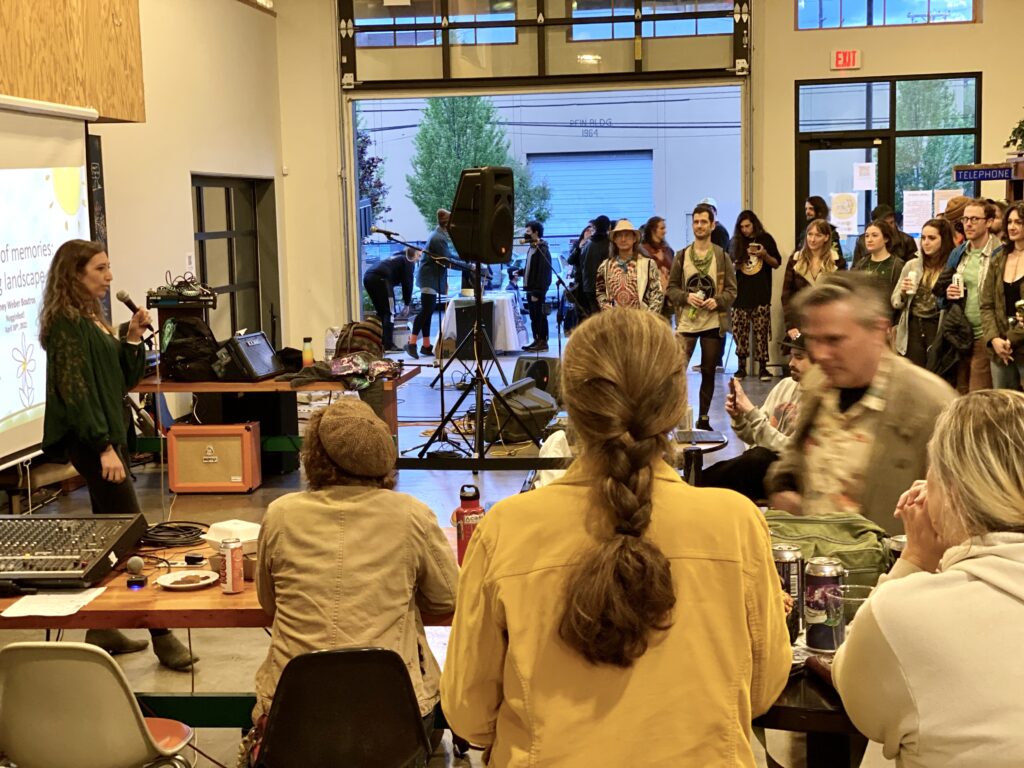
I want you to picture your last vacation…
Really picture it
When was it?
Where did you go?
How did you get there?
Who was with you?
What were you feeling?
What were you smelling, or tasting, or hearing?
While I would love to stay in “vacation mode,” come back to the present with me.
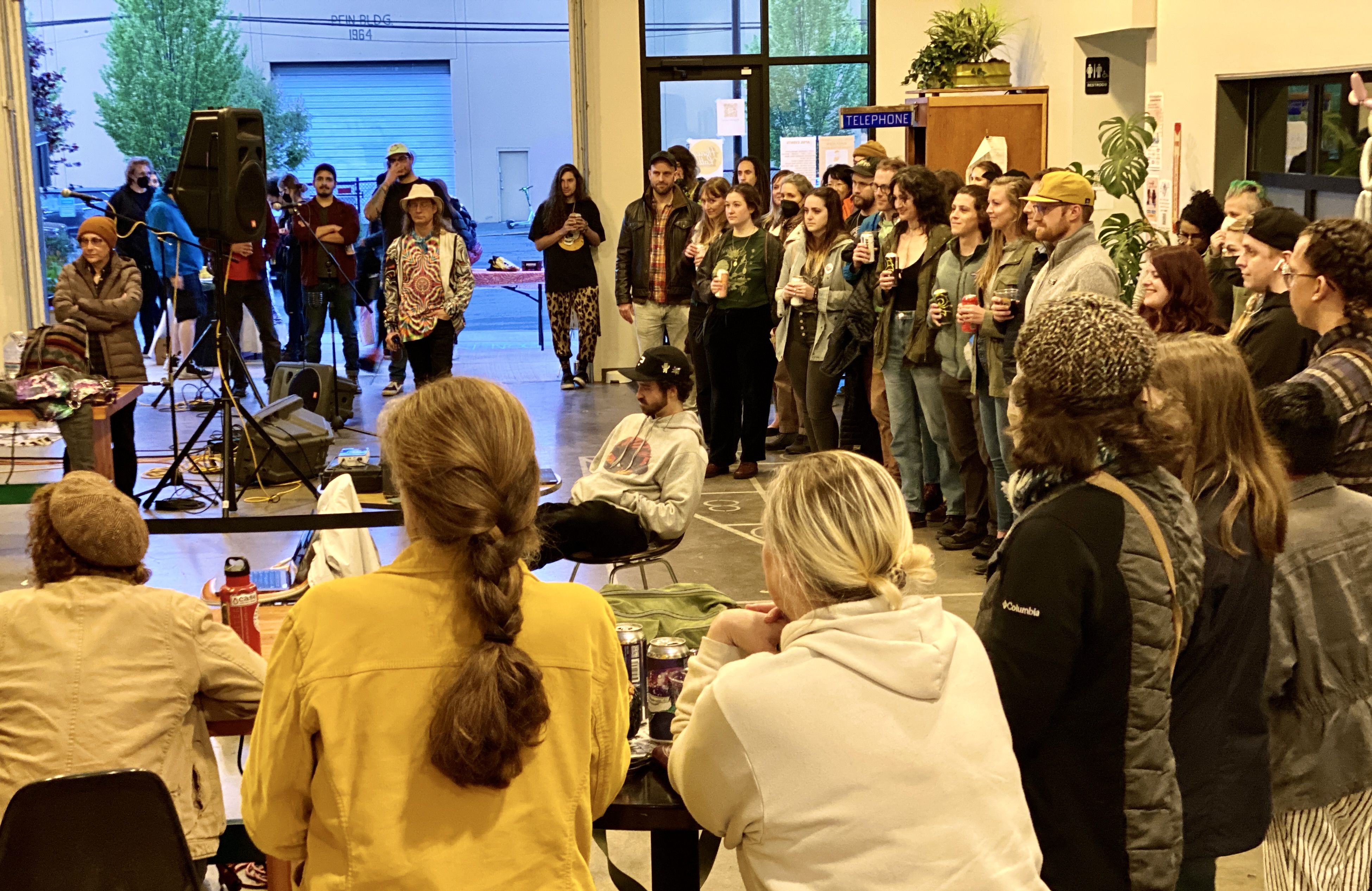
I just asked you to participate in a specific type of memory recall — you remembering your last vacation is a type of memory we call “explicit” or ”declarative,” because this type of memory requires conscious effort. I asked you to reflect on some different pieces of your explicit memory, which we can divide into “episodic” or “semantic” memory. Episodic memory is highly personal — it is anything considered autobiographical, like what you were feeling, what you were hearing, seeing, tasting — all the pieces of your memory that are unique to you. Semantic memories are memories that are factual — like where you vacationed, when you went, how you got there — all the pieces that anyone could know.
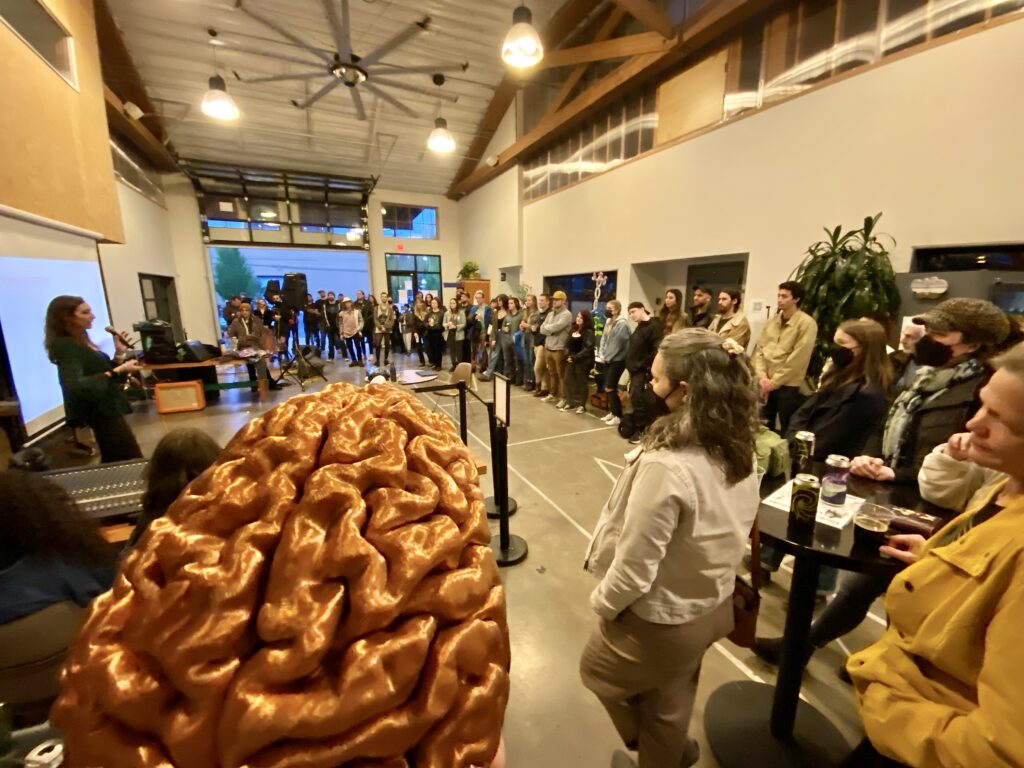
LEARN MORE: What are the differences between long-term, short-term, and working memory?
There is another major type of memory: “implicit” or “nondeclarative,” which does not require conscious effort. There are 3 major sub-categories of implicit memory: Associative, Priming, and Procedural.
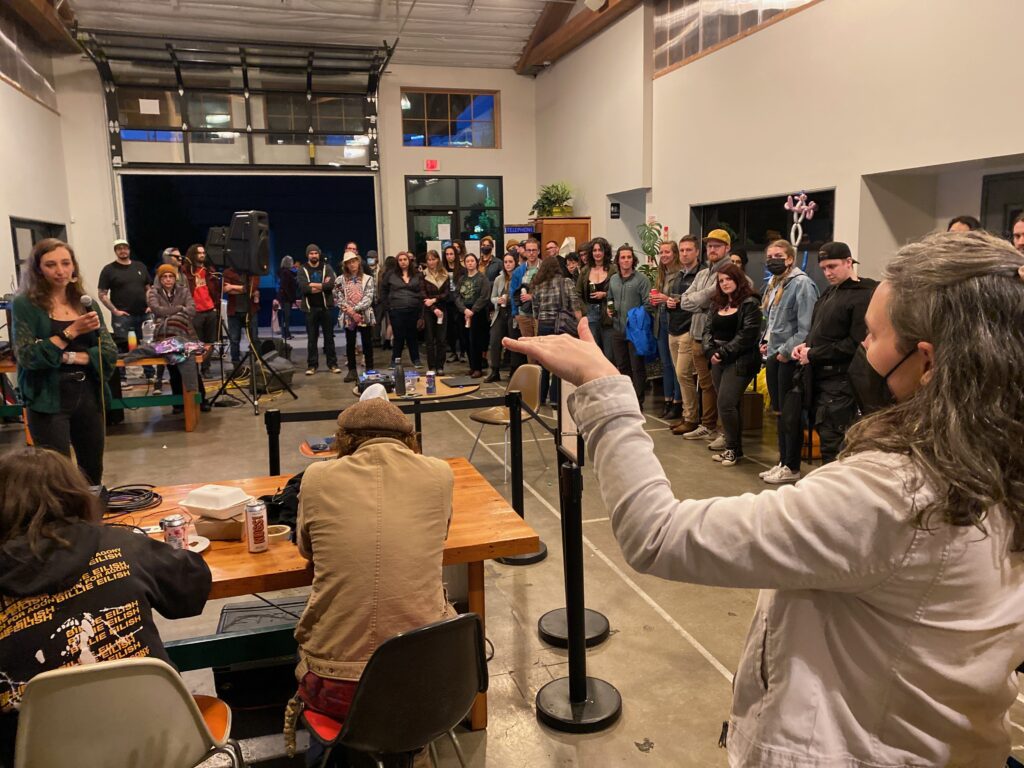
You may have heard about Dr. Ivan Pavlov and his dogs that learned to salivate at the sound of a bell – or you may have a dog who learns that a “click” is followed by a treat. This is Associative learning, the pairing of stimuli.
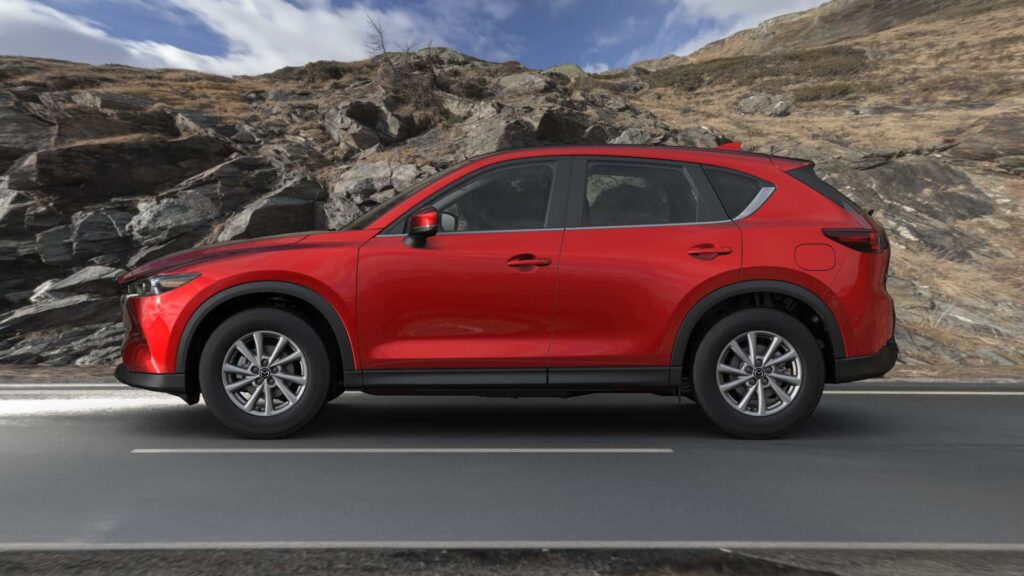
Priming has to do with “nearness” of concepts. You may notice that there are a lot of Red Mazda CX5 cars on the road right after you buy a Red Mazda CX5. I’m going to break it to you that there are not more of these cars, you are simply noticing them more because you are primed by your recent experience.
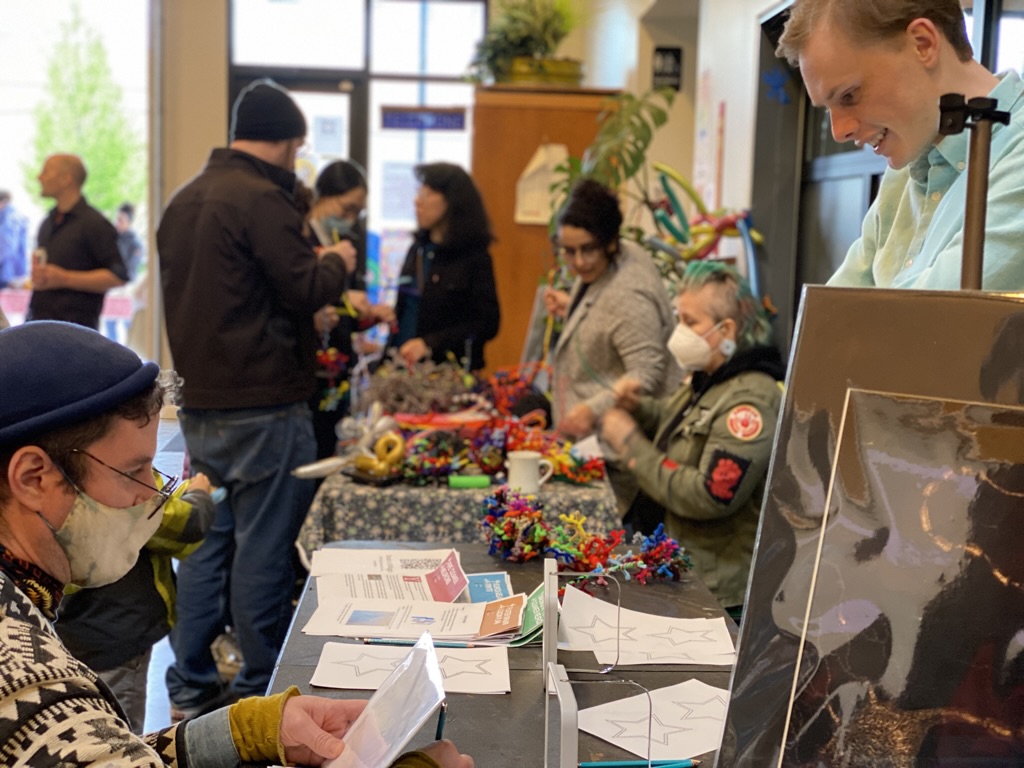
Procedural is the activity that we set up here at NogginFest, at our “mirror drawing” table. Mirror drawing is an example of “motor memory”; riding a bike, throwing a ball and writing are all examples of procedural memory. The distinctions between these types of memory are not superficial – these abilities are dependent on different brain regions, which we know from decades of neuroscience research.
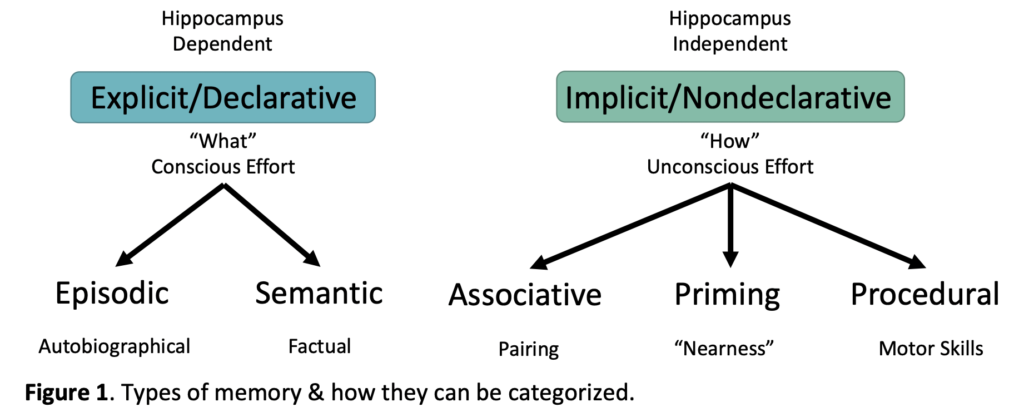
LEARN MORE: The Legacy of Patient H.M. for Neuroscience
LEARN MORE: Brain Systems Underlying Declarative and Procedural Memories
LEARN MORE: Conscious and Unconscious Memory Systems
But, I want to focus on the similarities of the learning process and how we get to actually planting and cultivating a memory.
Right now, every single moment all the time, information is hitting us. There is NO possible way we can process all of the stimuli around us – it is simply too much. We call this the “sensory stage,” because the information is there, we are sensing the wind in the trees in the background, the draft coming from the window, but most of this sensory information is going to wash over us. This is like throwing handfuls of wildflower seeds into the air, we’re going to lose track of where they end up.
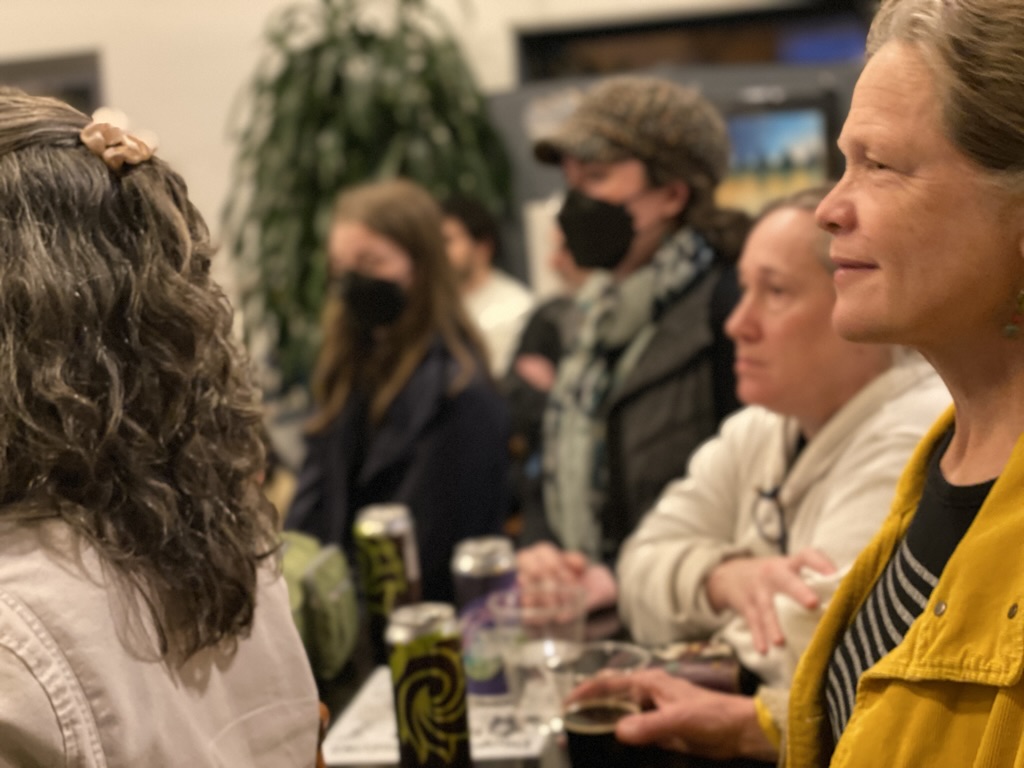
UNLESS we turn our attention to the smell of those jasmine flowers, or the people talking in the other room. Attention is the first step in transitioning to the short-term stage. This stage is taking a portion of seeds and intentionally planting them into the dirt-–we are focused on this. But, we have a limited capacity. We can’t possibly attend to hundreds of individual seeds. We have the capacity to attend to around 7 (give or take a couple) at a time, and – as the name implies – only for about 30 seconds.
UNLESS we rehearse this information.
Rehearsal is like placing stakes in the ground next to the seeds we decided to attend to. By repetition or effortful indication, information gets stored into the long-term stage, which has no capacity or time limits. Getting information into this stage presumably stays with us forever.
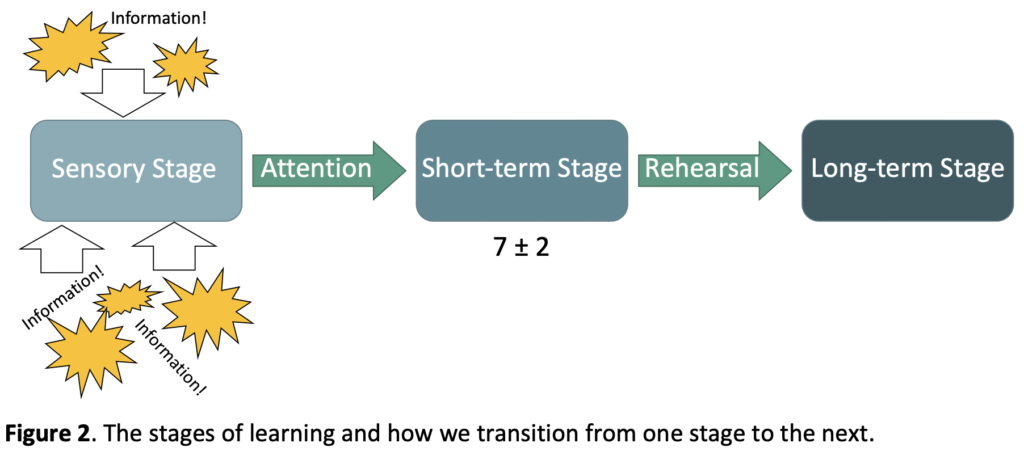
I like to think of all of this as the planting process, getting information into the soil to grow. But there are a lot of steps to cultivate and grow memories.
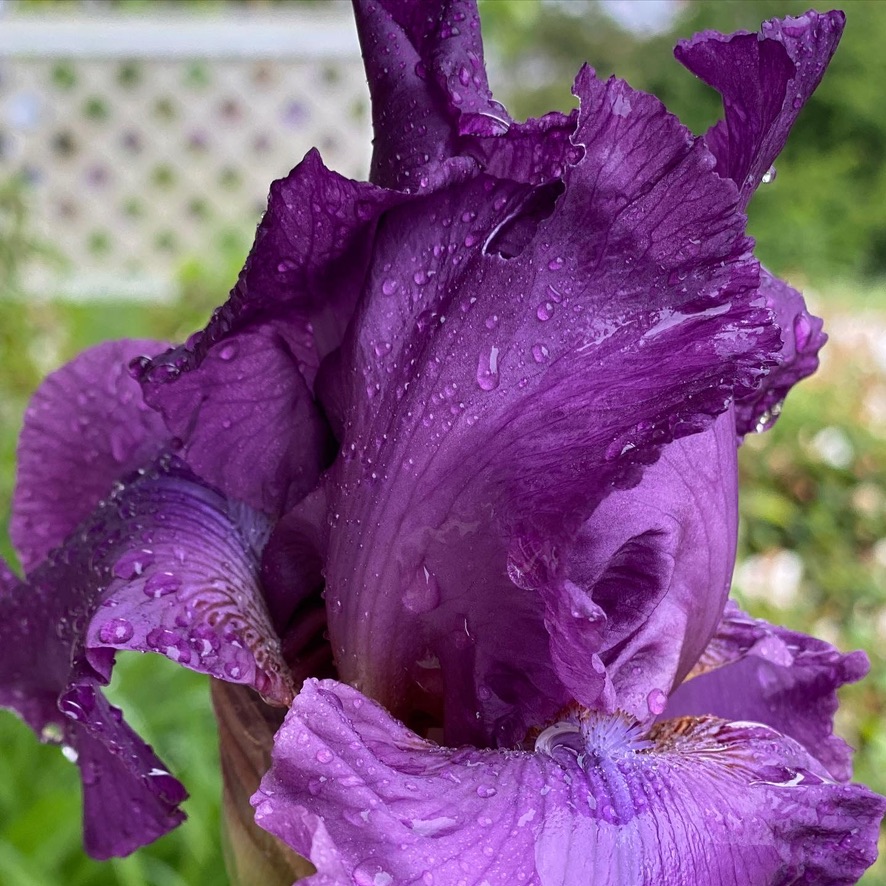
Long term potentiation (LTP)
When we think about growing memories, we need to dive into the brain a bit. Specifically, to a concept known as “Long Term Potentiation” or “LTP.”
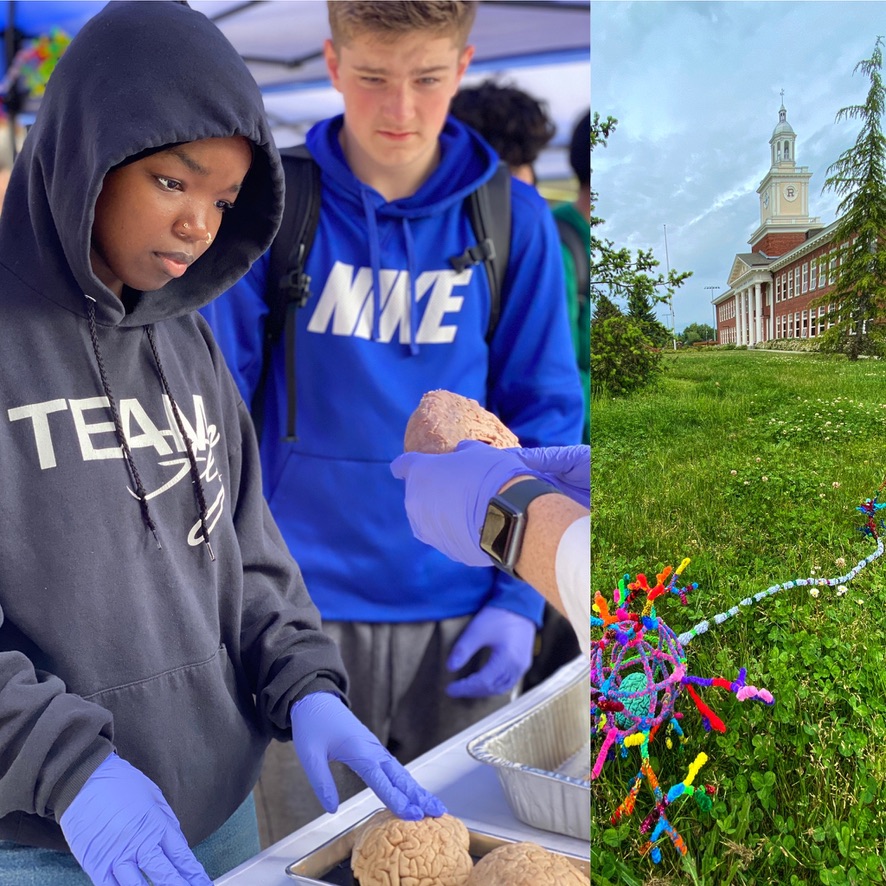
In figure 3, we are looking at my own depiction of neurons, the primary cell type of the brain.
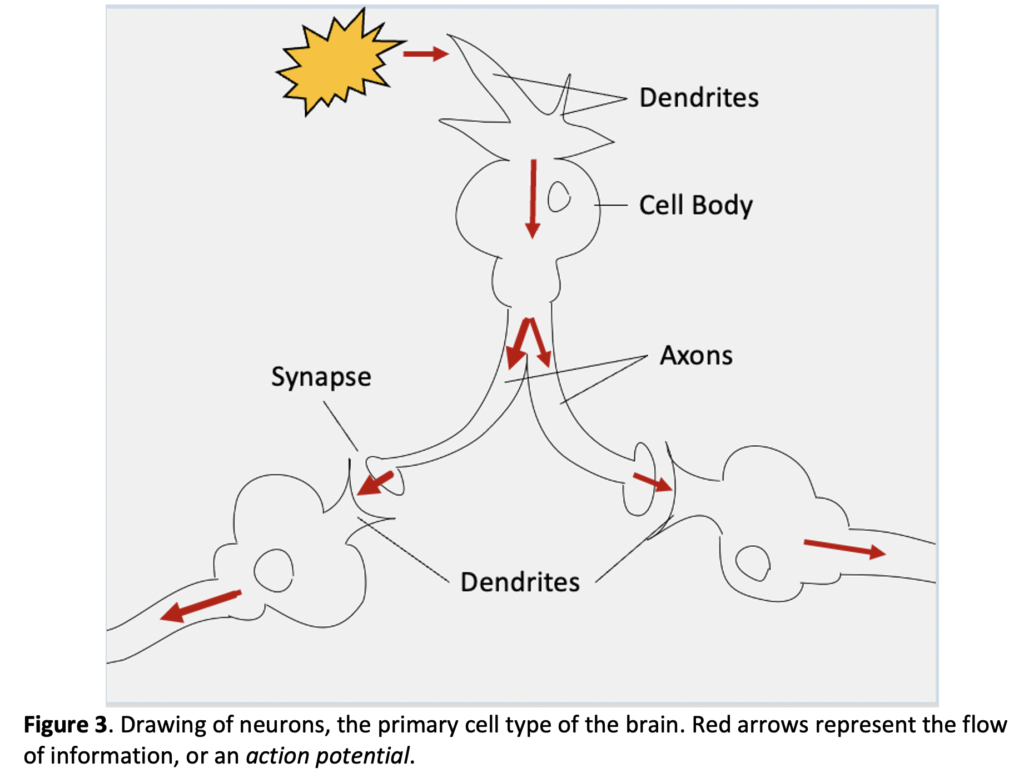
Neurons communicate a lot, and there are a few key pieces that facilitate communication. Dendrites receive signals. When something important happens, like seeing a picture of a neuron, and you turn your attention to it. This external stimulus will initiate an action potential, the “message.” This message will continue through center, called the cell body, or the control center of the cell. The message will continue down the neuron’s axons, which are responsible for sending signals.
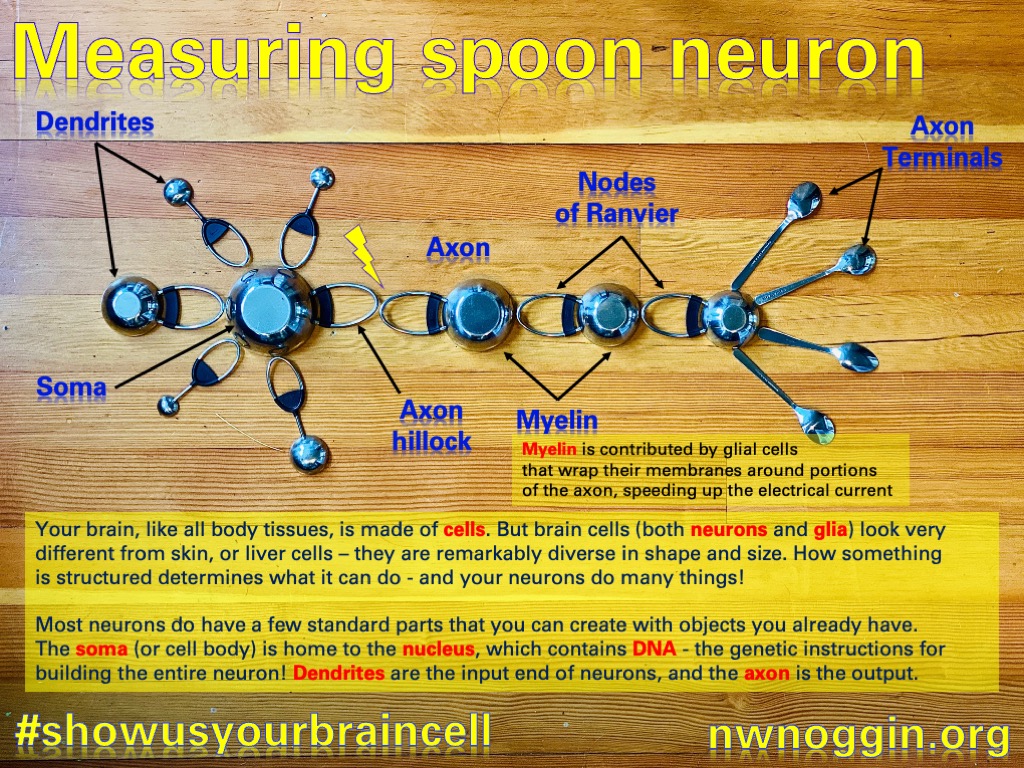
The signal is communicated to the next cell via a gap called the synapse, and the dendrite of the next neuron picks it up to continue on the message.
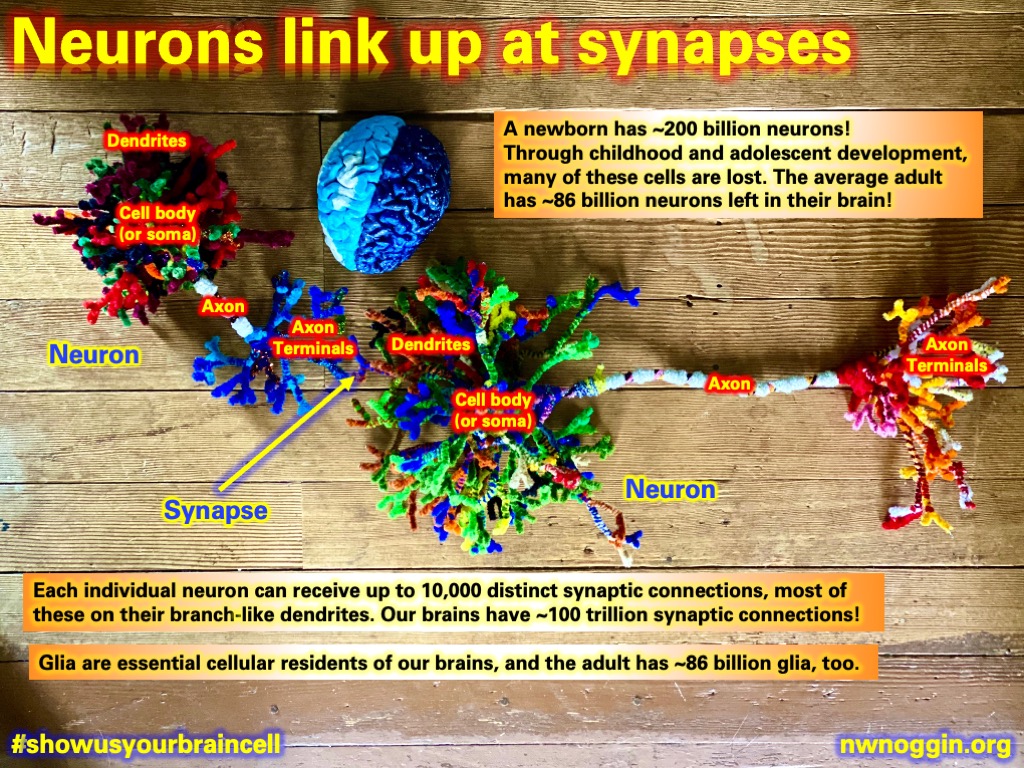
Importantly, there is a lot of specificity in this signaling, which is where “LTP” comes in to play. During learning, when we are attending and rehearsing information, there is increased communication between cells that are important for this information and decreased communication between cells that are not important.
You may have heard the phrase “cells that fire together, wire together.”
LEARN MORE: Fire Together, Wire Together: Carla Shatz on Scientific Breakthroughs
With repeated firing over time, our neurons actually grow and change, where we see new axons, synapses, and dendrites sprouting between these cells to facilitate more efficient flow of information. This takes a lot of energy, and like in our gardens, we want to channel as many resources we can into growing connections that are important – one way we can do this is by pulling weeds.
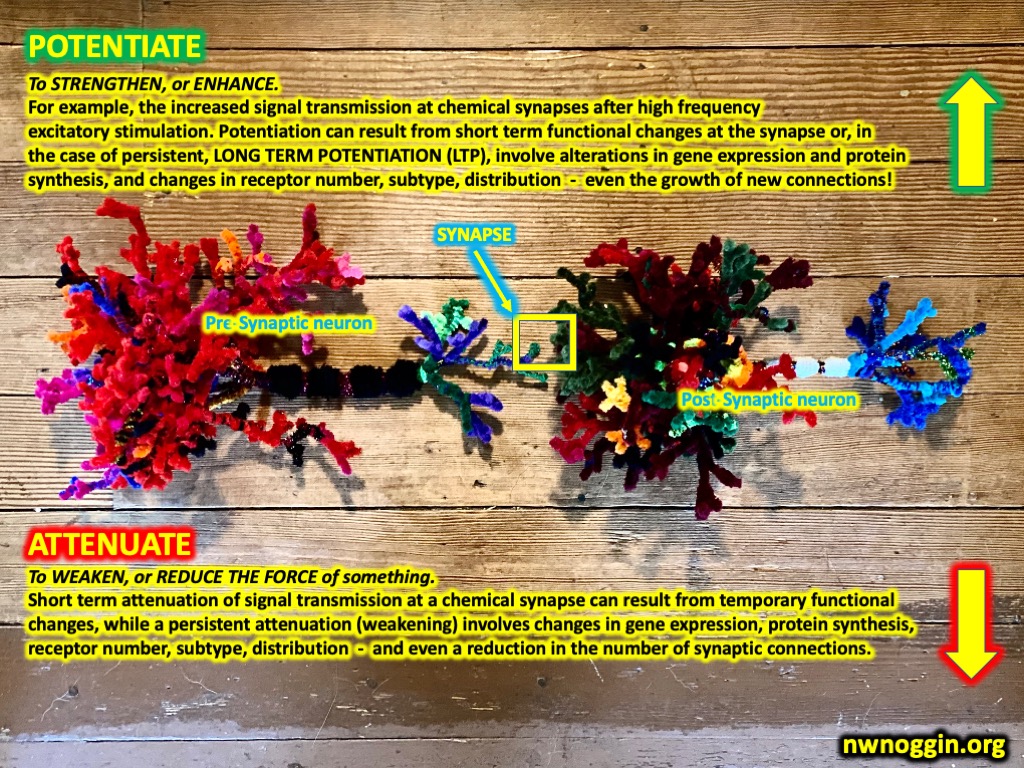
So, in addition to this increased communication, we also see pruning of synapses that are not carrying meaningful information.
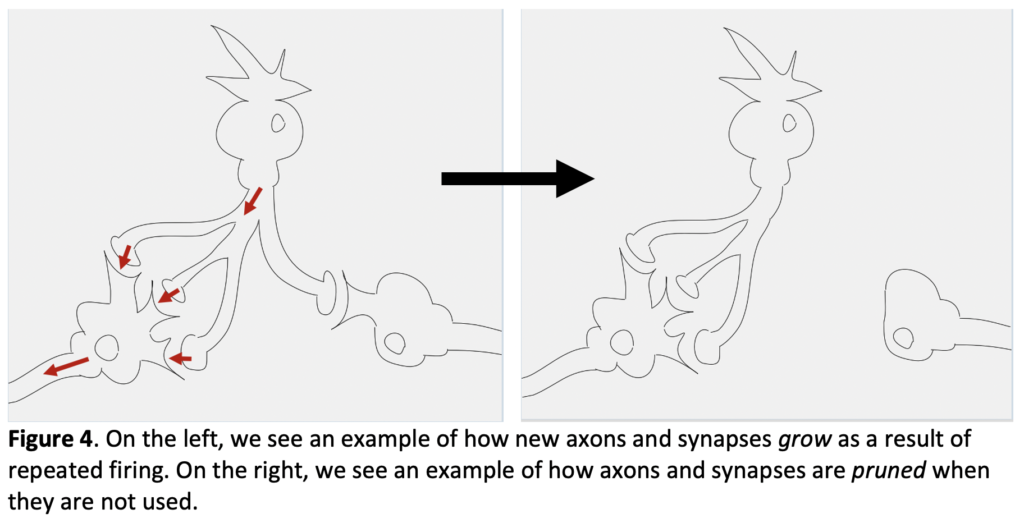
Who is the “gardener?”
I want to emphasize that this growth and pruning of axons is so dynamic – this is happening in our brains all the time. One big question in the field has been what is the process that leads to this, or, in other words, who is the gardener?
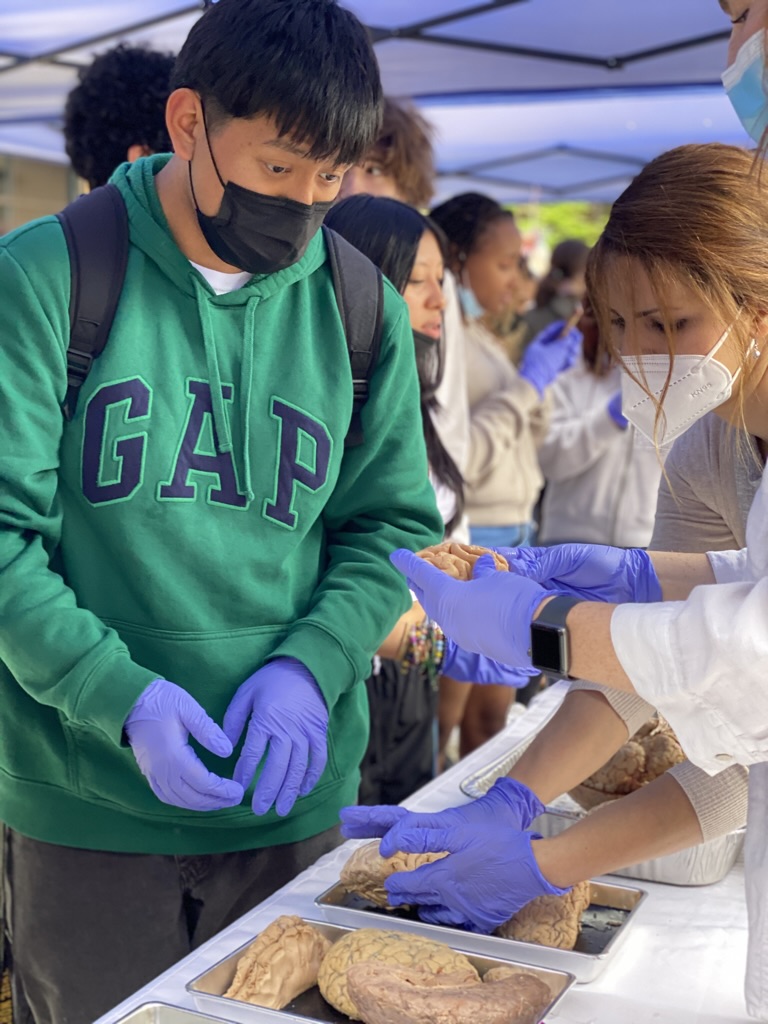
What is causing this growth and pruning to happen – it’s not like we are sitting here telling our neurons “ok grow a new axon in this direction, and let’s prune this other axon over here.” Which brings us to the central dogma of biology: our DNA transcribing to RNA, translating to proteins.
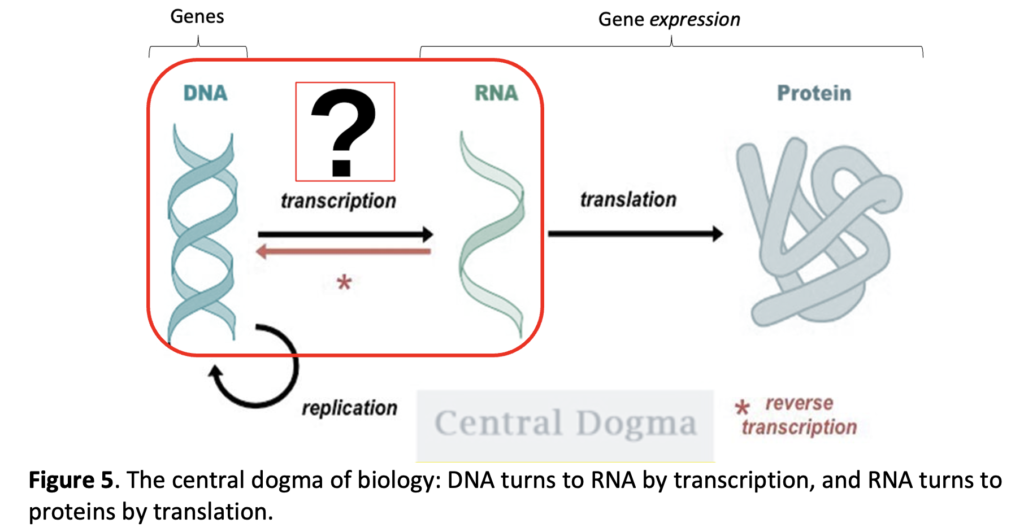
In our bodies (including our brain), DNA is the architect of everything. It is the blueprints to our being. RNA are like the project managers—they receive the blueprints from the architect DNA, and take that information to the contractors, our proteins. Proteins actually do the work, like building new synapses. The information is held by the DNA, but proteins are responsible for executing the orders.
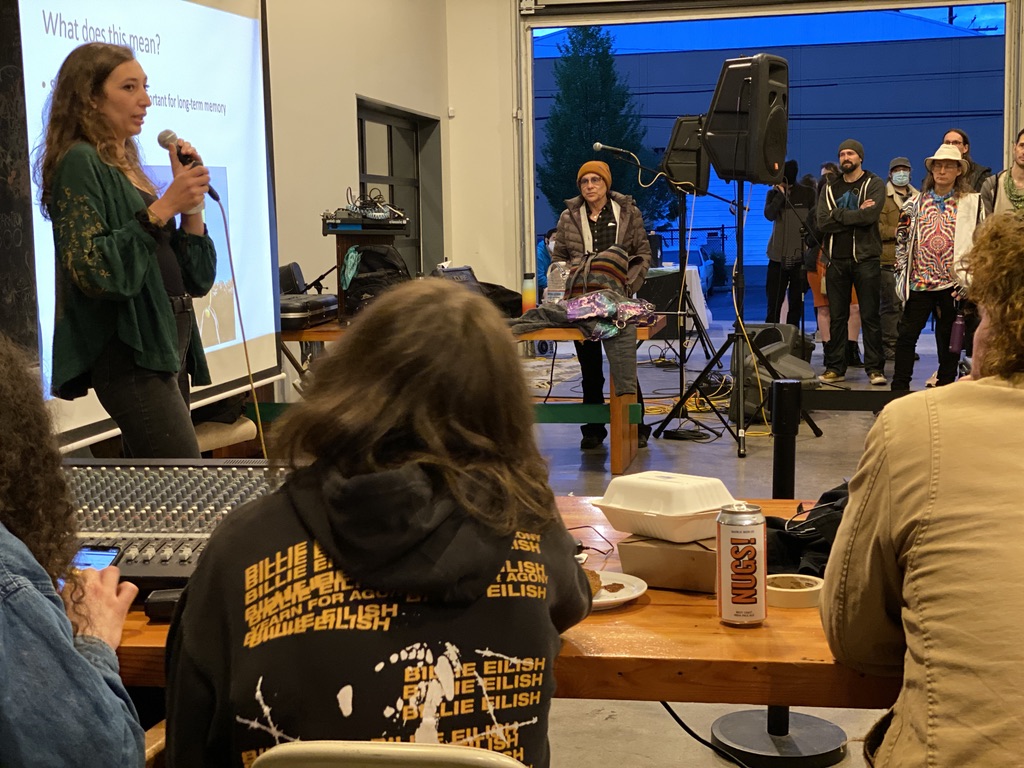
My work has focused on an important step in this process—how does the DNA to RNA transcription happen during learning, and how does it happen quickly?
Breaking DNA
It’s important for the information to be shared from DNA to protein rapidly in order to accurately learn and remember. Which leads to the driving hypothesis of my research: that DNA breaks in order to induce gene expression, which contributes to long-term memory.
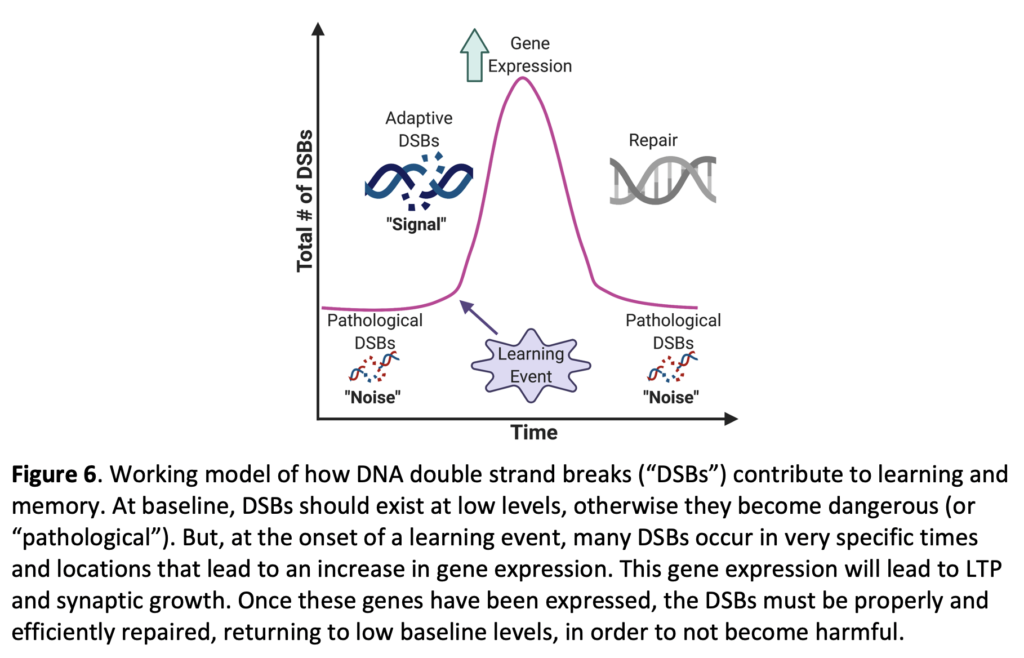
To test this hypothesis—that DNA double strand breaks (DSBs) contribute to learning—I chose to manipulate DSBs in a brain region known to be important for learning and memory: the hippocampus. I manipulated DSBs with a drug called etoposide, which is used to treat cancer daily. Etoposide increases DSBs in the body, but I chose to directly target the hippocampus.
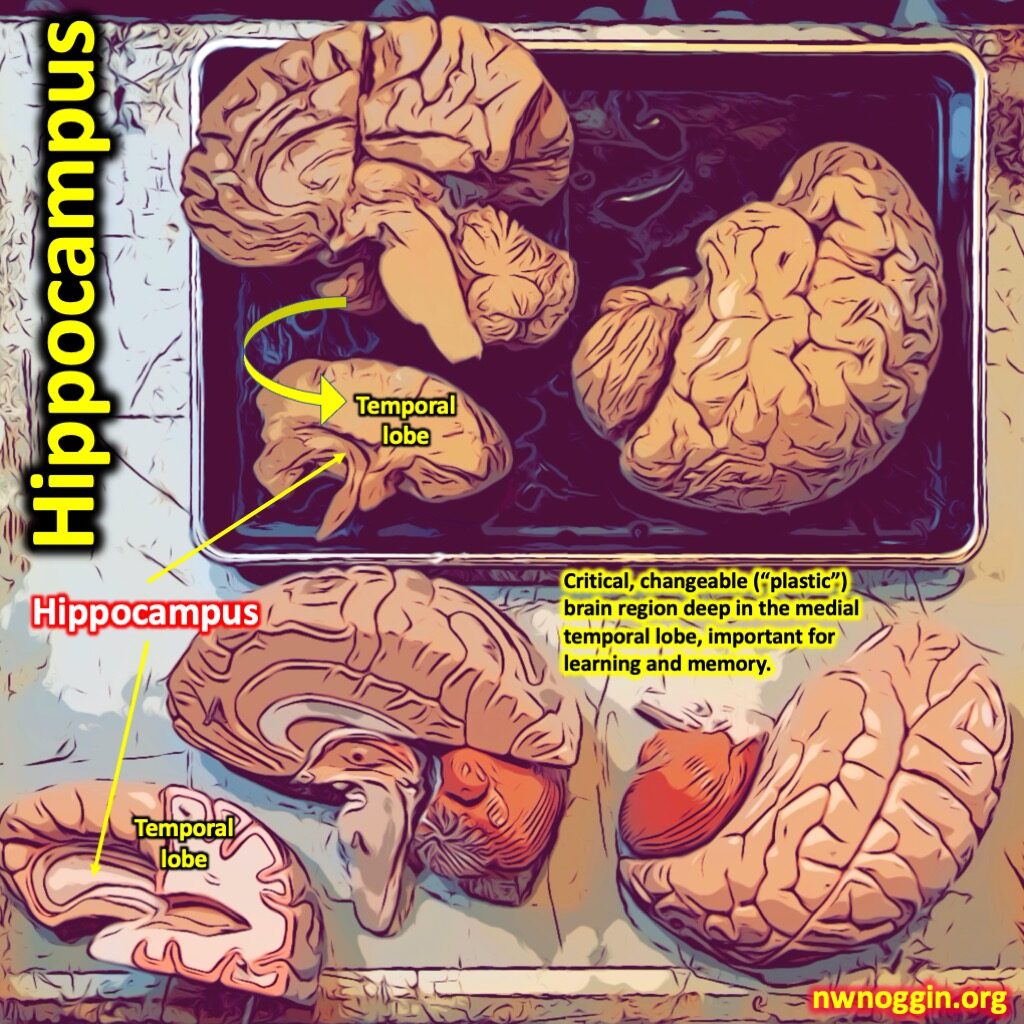
I performed aseptic surgery on mice to place ports into the CA1 region of their hippocampus. After they fully recovered, I infused saline (control) or etoposide directly into the hippocampus, then trained them in a test called fear conditioning. During fear conditioning, the mice received 4 mild foot shocks paired with a specific tone (a cue). The animals should learn two things: 1) that the box where they receive the shocks is a dangerous place (the context), and 2) that the tone is a dangerous cue.
Importantly, contextual memory is dependent on the hippocampus, while cued memory is dependent on a different brain region called the amygdala.
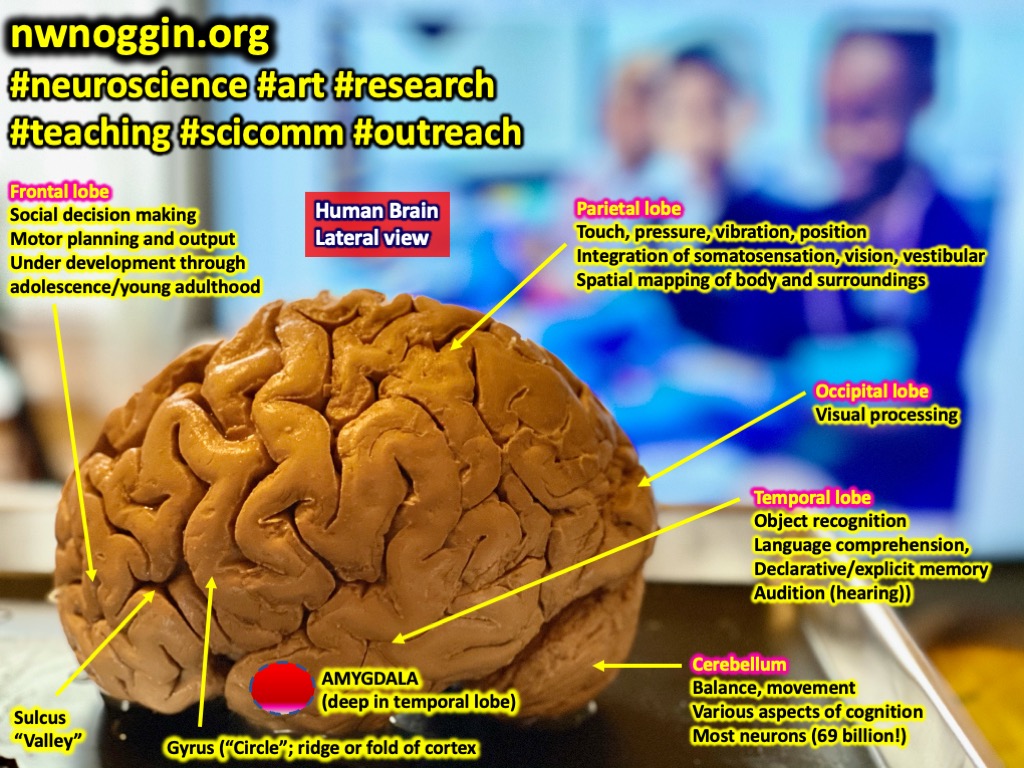
After training the animals in fear conditioning, I can ask if they remember the context by placing them back into the same box and if they remember the cue by placing them in a different box but play the same tone. I then measure how much time they spend freezing as a measure of their memory. By infusing etoposide only into the hippocampus, I predicted that mice would freeze less during the contextual tests (impaired memory) compared to saline-infused mice, but that all mice would freeze similarly in the cued tests.
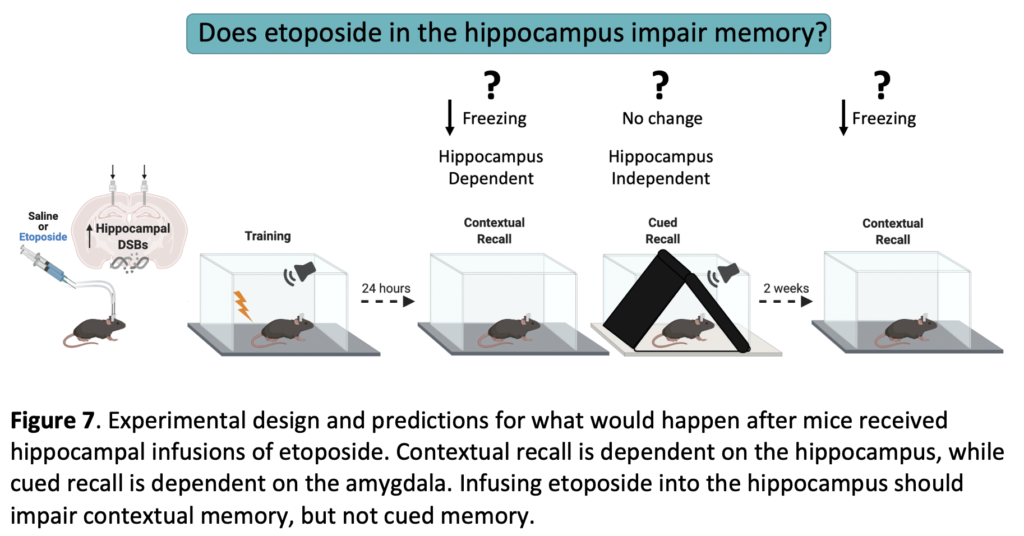
When I conducted these experiments, I was very excited to find that etoposide infusions into the hippocampus did impair contextual memory! Both 24 hours and 2 weeks after the initial training, mice that received etoposide froze less than mice that received saline, telling me that they had a worse memory for the context. Looking at the hippocampus, I also found that there was less gene expression (of a specific gene called cFos) in the hippocampus of mice that received etoposide. Importantly, all mice froze the same amount in response to the cue, telling me that there was no impairment for a hippocampus-independent memory.
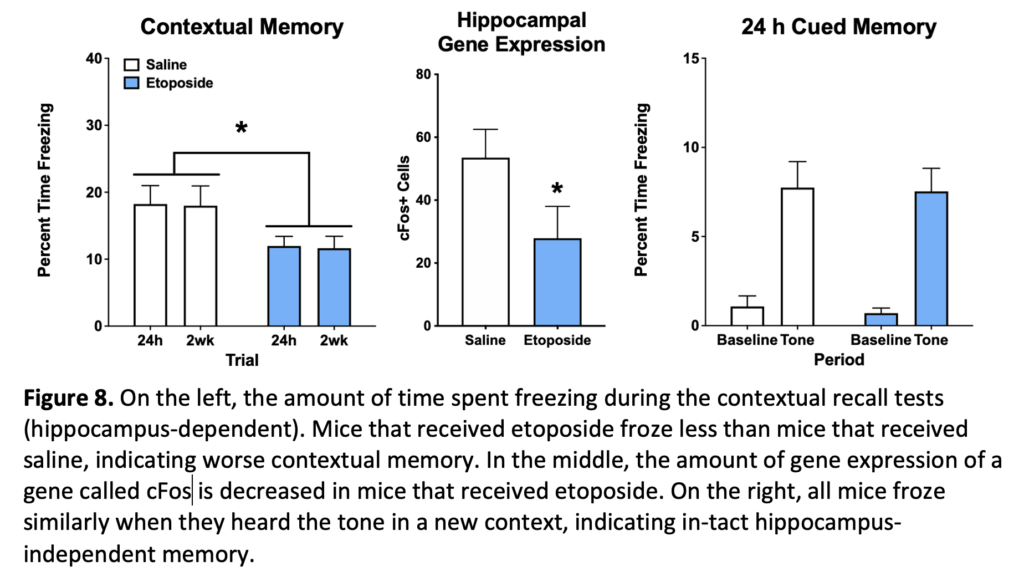
What exactly does this all mean for daily life?
My data suggest that DNA breaks are important for proper learning and memory to occur.
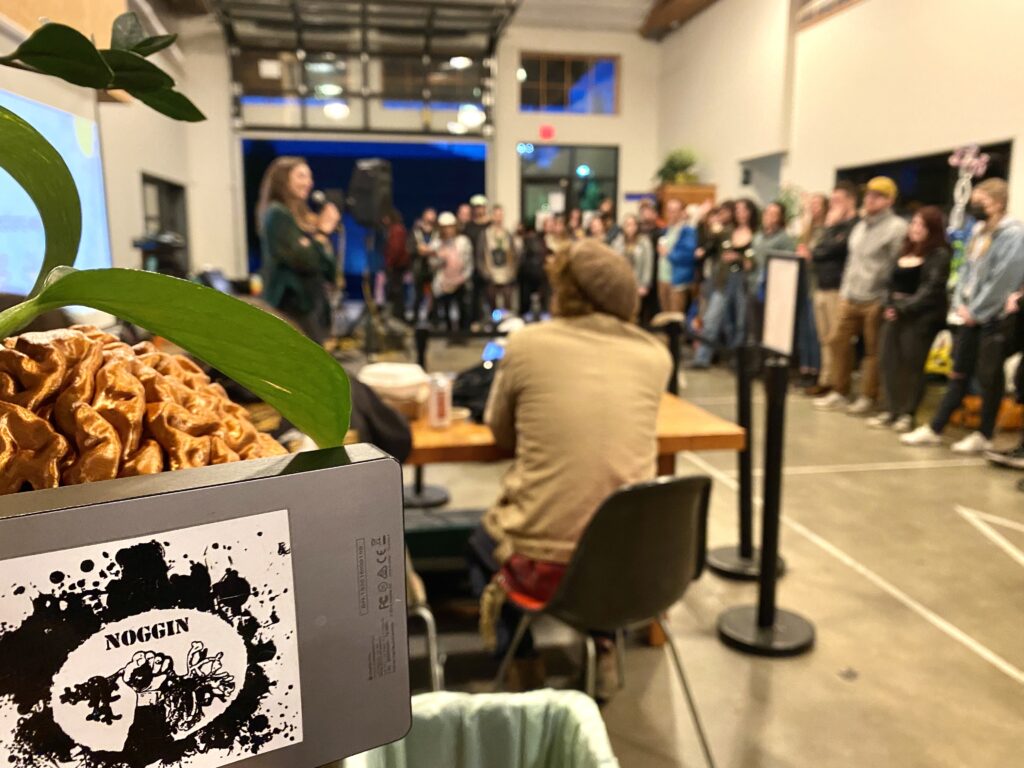
I find that this is similar to seeds cracking in order to sprout roots—without a break in the seed, the growing process cannot begin. These data also suggest that dysregulation of DSBs could contribute to a range of learning-related disorders, including ADHD and Alzheimer’s disease. Current treatments for learning-related disorders (especially neurodegeneration) are not great, and by taking a look at this new mechanism, we might be able to develop new and better treatments to stop the pain and struggle of those afflicted by them.
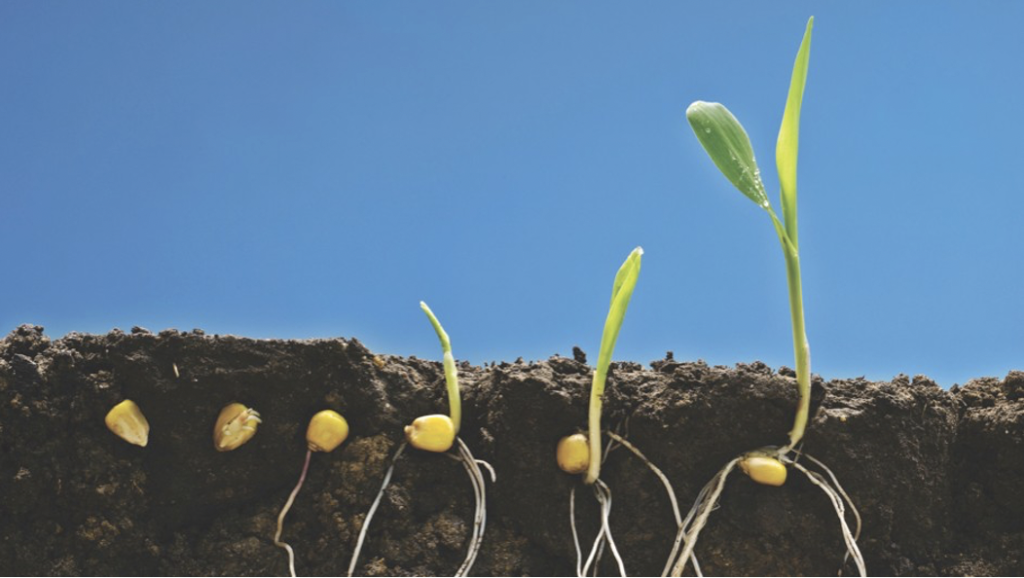
“We do not inherit the earth from our ancestors, we borrow it from our children.”
– Chief Seattle of the Suquamish and Duwamish Tribes