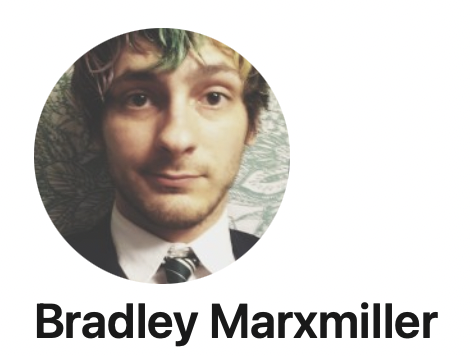
Post by Bradley Marxmiller, undergraduate in Biology and Interdisciplinary Neuroscience at Portland State University. Bradley also wrote this introductory post: How do you find a job in a lab?
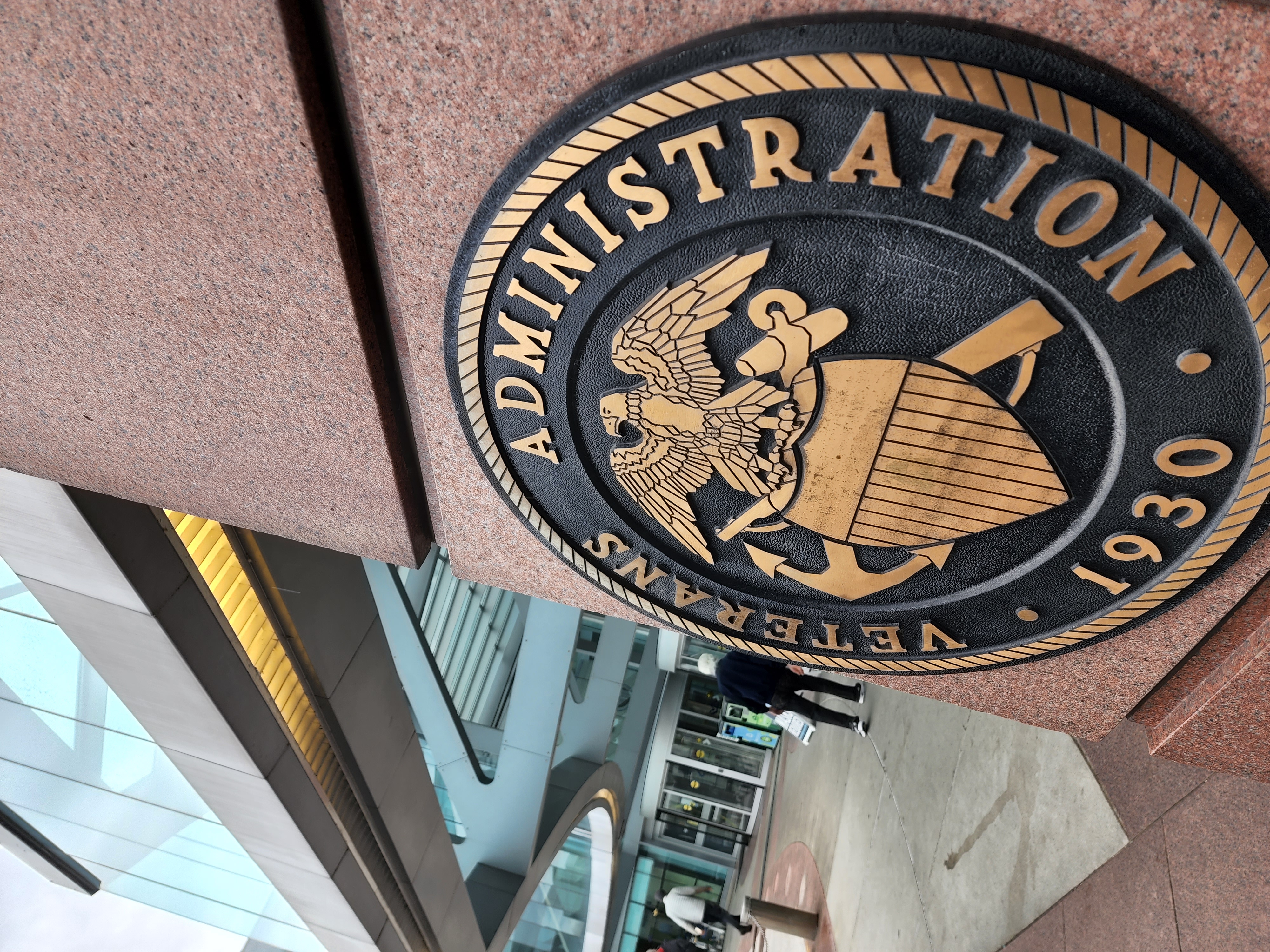
When I finally secured funding and a position doing neuroscience research, it felt like a huge accomplishment. However, more than a goal or accomplishment, it is really an amazing opportunity to be a part of a collaborative scientific community that is doing the hard work of solving some of the world’s oldest questions: why do we think the way we do?
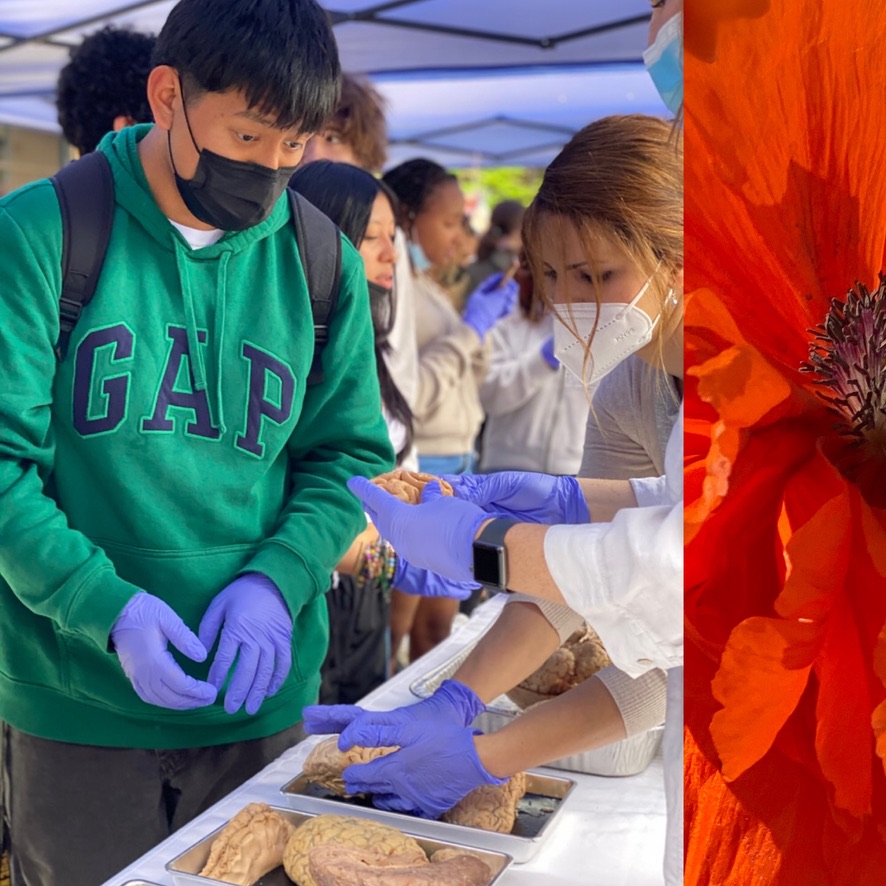
Much of the earliest of the modern neuroscience knowledge comes from unfortunate accidents, tumors, or other forms of damage to the brain. Scientists note changes in behavior or function as a result of that damage and reverse engineer a map of neural function by looking for the location of the damage. A famous case is that of Phineas Gage: who lost part of his frontal lobe in an occupational related accident and experienced a loss of impulse control, amongst other alterations to his personality.
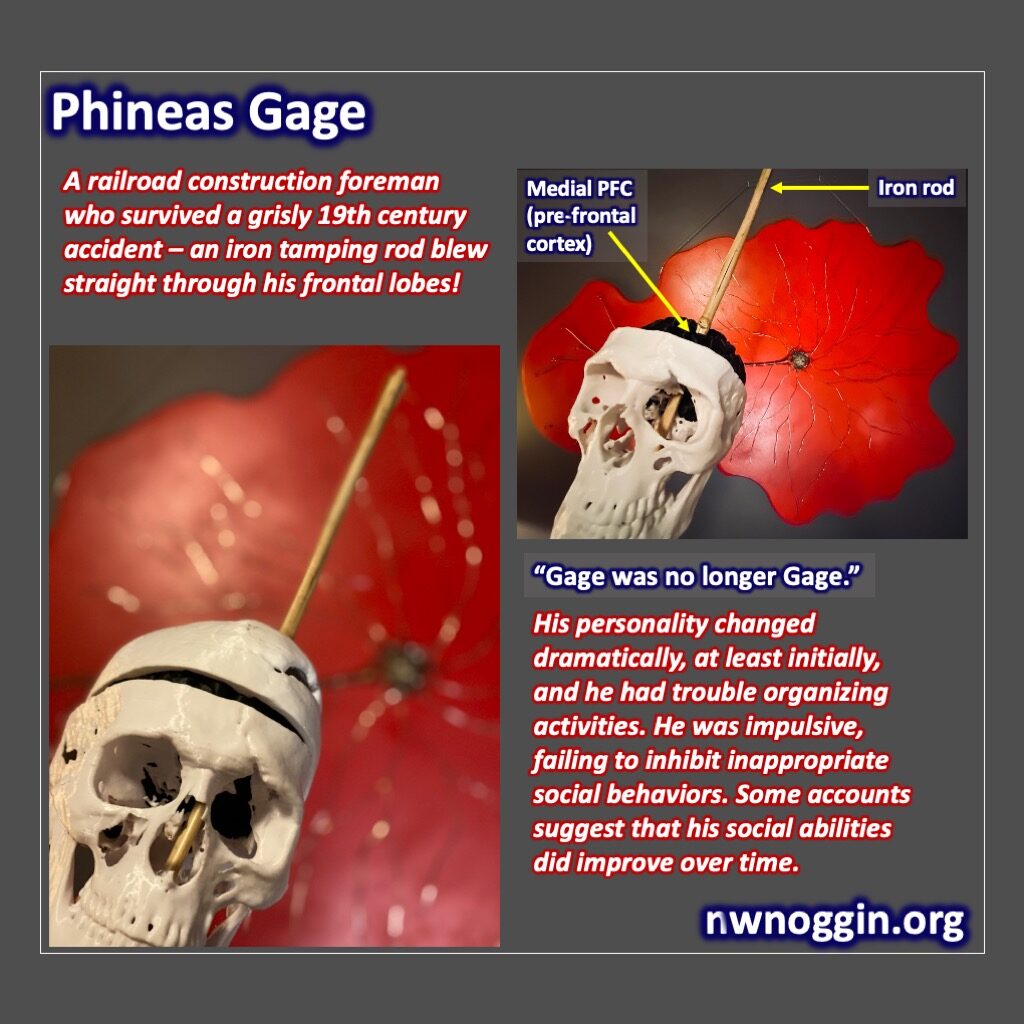
LEARN MORE: Franklin and Phineas Gage
LEARN MORE: Phineas Gage’s great legacy
However, it’s unlikely that we will map every area of the brain’s functions by random chance. Therefore, the modern era has brought noninvasive imaging techniques which have been great for the general mapping of brain function. Working with human’s and imaging techniques with functioning magnetic resonance imaging (fMRI) and electroencephalograms (EEG) has given us a rough draft map of the brain.
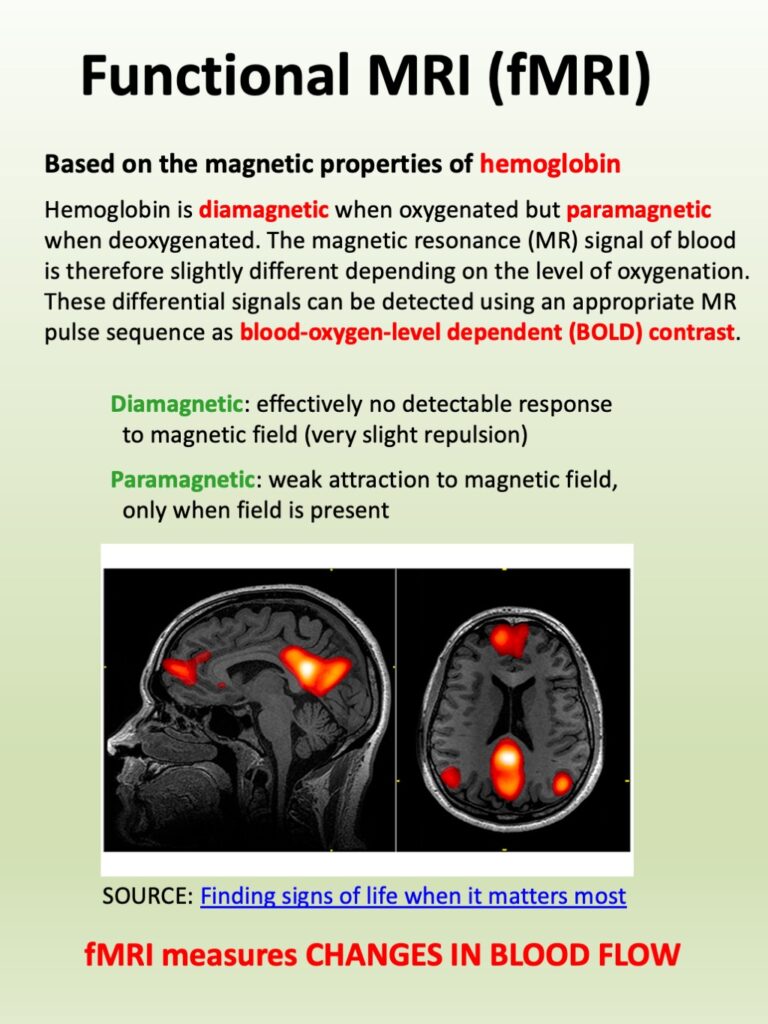
LEARN MORE: Overview of Functional Magnetic Resonance Imaging
LEARN MORE: Electroencephalography (EEG)
Unfortunately, these techniques still haven’t answered a lot of questions about brain function. Low temporal (time) resolution (neurons can fire hundreds of times per second) leaves questions about the timing of events in the brain in fMRIs, in addition to the expense of running the machine; and low spatial information from techniques like EEGs leave questions about exactly where fast spiking brain activity is happening. To get the timing and spacing of brain functions more accurately, invasive implants are one of the cheapest and most effective solutions. However, surgical implantation of electrodes in humans would be unethical except in extremely rare situations, and it would take a long time to get enough information or develop better techniques based on only a few cases. Instead, when there is sufficient evidence that an area of the brain that has homology (has a locational or genetic similarly) in an animal it is much more ethical to model the brain in mice or primates while developing targets for medications or further advancing our understanding of brain regions.
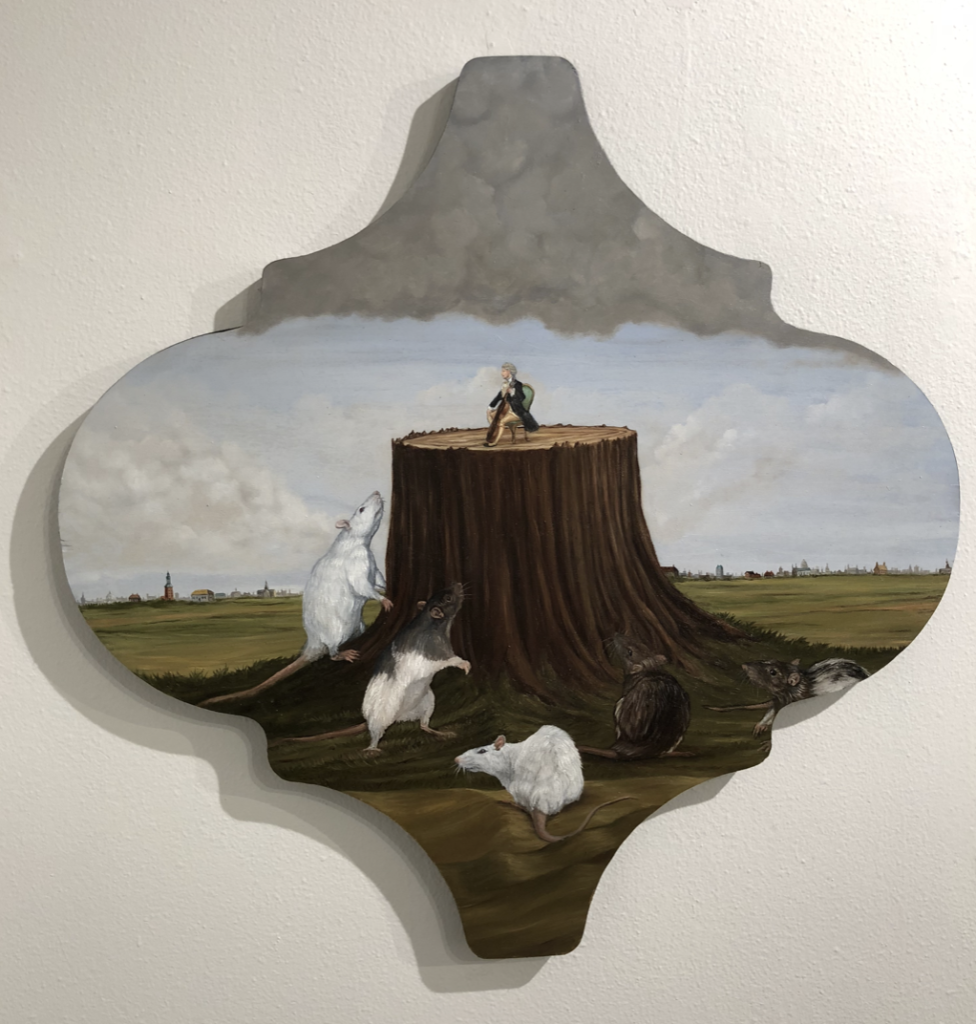
Painting by Jeff Leake
LEARN MORE: Human Subjects in Research: Things to Consider
LEARN MORE: The Emergence and Development of Animal Research Ethics
While these techniques have been extremely helpful, further advances in evolutionary and genetic sciences have allowed us to develop an even better understanding of smaller circuits (interconnected areas of the brain). Namely, we have so-called “knockout” mice which use the similar principles as our learning about personality from Phineas; we remove the genes for one type of neuron or another and watch the developmental and behavioral changes that occur. However, it is still hard to demonstrate that the particular differences we see are directly because of those changes. It could be that natural differences between a line of mice for example are completely unrelated to the part we manipulated: perhaps something downstream that naturally evolved changed as well. To overcome this we have combined genetic technology and our knowledge of neural cell biology to create optogenetics.
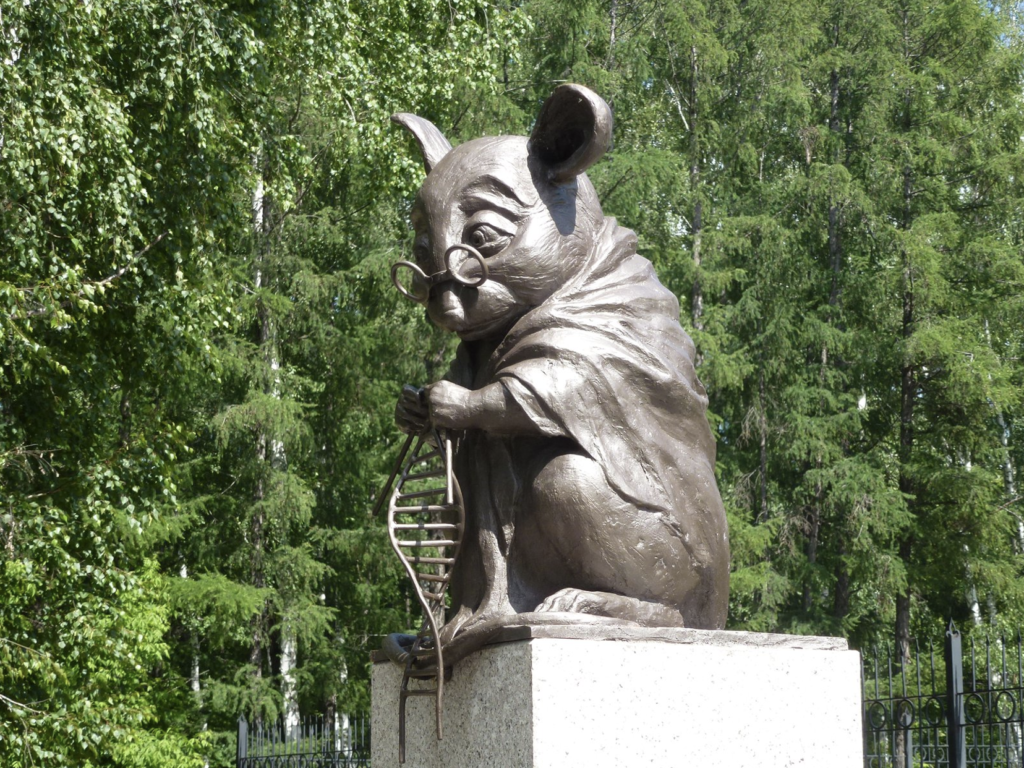
LEARN MORE: This Russian Monument Honors the Humble Lab Mouse
See the Light
Many people know nerve cells fire when the cells get enough input from sensory cells or other connected neurons. Those signals are communicated to the cell by small positively charged particles flowing into the cell, often through the release of a neurotransmitter. Neurotransmitters are chemicals that connect onto little gates on the target cell, prompting them to open and let the positively charged particles, causing them to fire(see video below). Alternatively, they might close gates, or open others that push positive ions out, to inhibit or prevent firing. With optogenetics, we have genetically installed special channel gates that react to a specific wavelength of light. This gives us the ability to silence or induce a discharge in a particular area or cell type.
In other words, we can view behavior and monitor connections when one type of brain cells in a limited area is turned on or off, giving us much more insight than just complete removal through accident, surgery, or genetic knockout.
“No one lights a lamp in order to hide it behind the door: the purpose of light is to create more light, to open people’s eyes, to reveal the marvels around.”
— Paulo Coelho
LEARN MORE: The Development and Application of Optogenetics
LEARN MORE: Optical Techniques in Optogenetics
LEARN MORE: Optogenetics in Neural Systems
At the Atheir Abbas Lab at the OHSU/VA, we utilize this technology to study the prefrontal cortex, the same area that was injured on Phineas Gage. However, we are looking at just one type of neuron within the cell, Vasoactive Intestinal Peptide (VIP) interneurons, instead of a wholesale removal of a large area. While the exact functions and activity of VIPs across regions are unknown to some degree (hence the need for research) they generally attach to other neurons and inhibit their firing by signaling to close gates that let in the positive ions (see further related readings below for more info). Adding a little more complexity, VIPs generally attach to other neurons that inhibit other neurons (they inhibit – or stop – the brakes on activity) – so they increase overall activity by largely limiting the activity of inhibitory neurons.
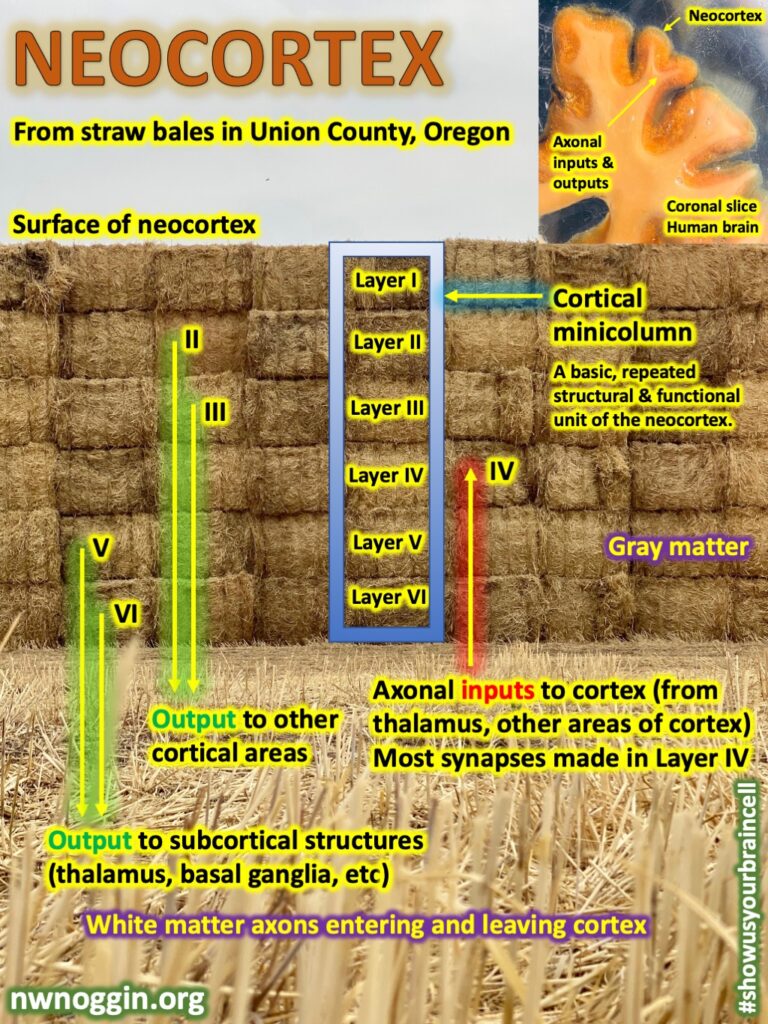

IMAGE SOURCE: VIP neurons in prefrontal cortical layers.
LEARN MORE: Inhibitory Neurons: Vip Cells Hit the Brake on Inhibition
To induce the growth of these modified channels we inject an Adeno-Associated Virus (AAV) that contains instructions for the cell to create light sensitive gates in the cells using the Archaerhodopsin (Arch) gene. The instructions to build Arch mediated gates are shown below in purple in the diagram of the AAV vector we use in the lab.
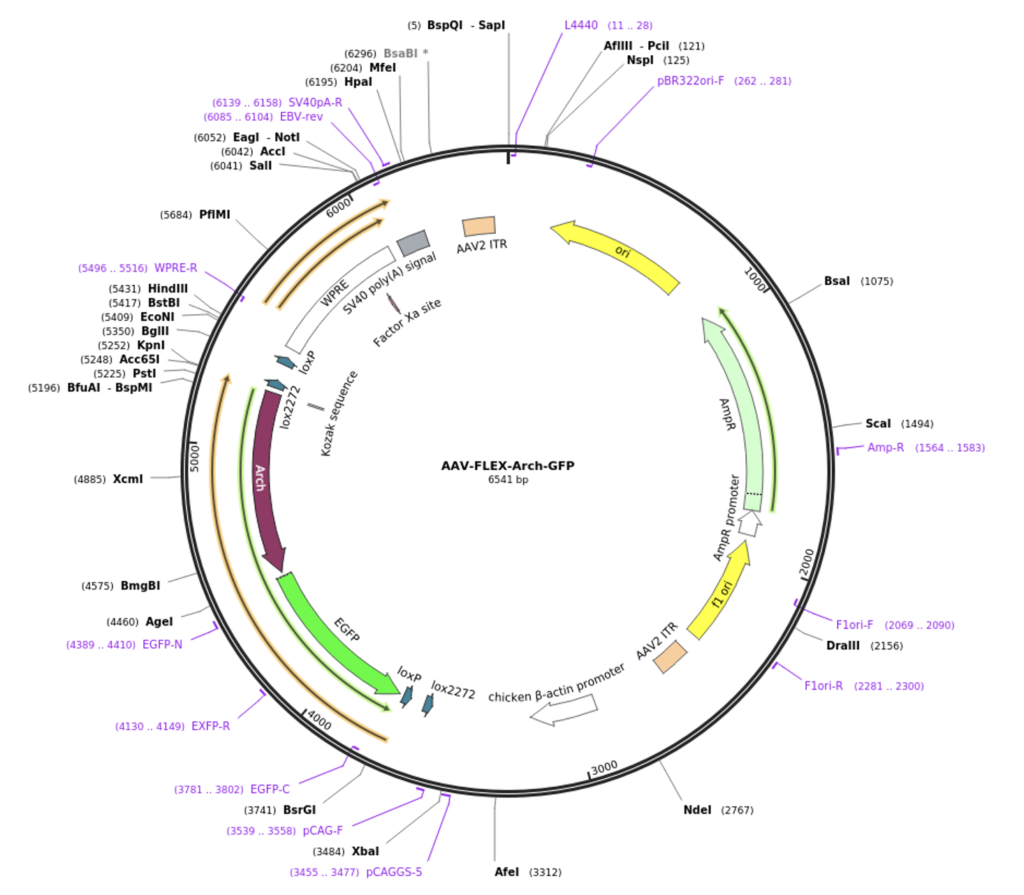
IMAGE: AAV-FLEX-Arch-GFP
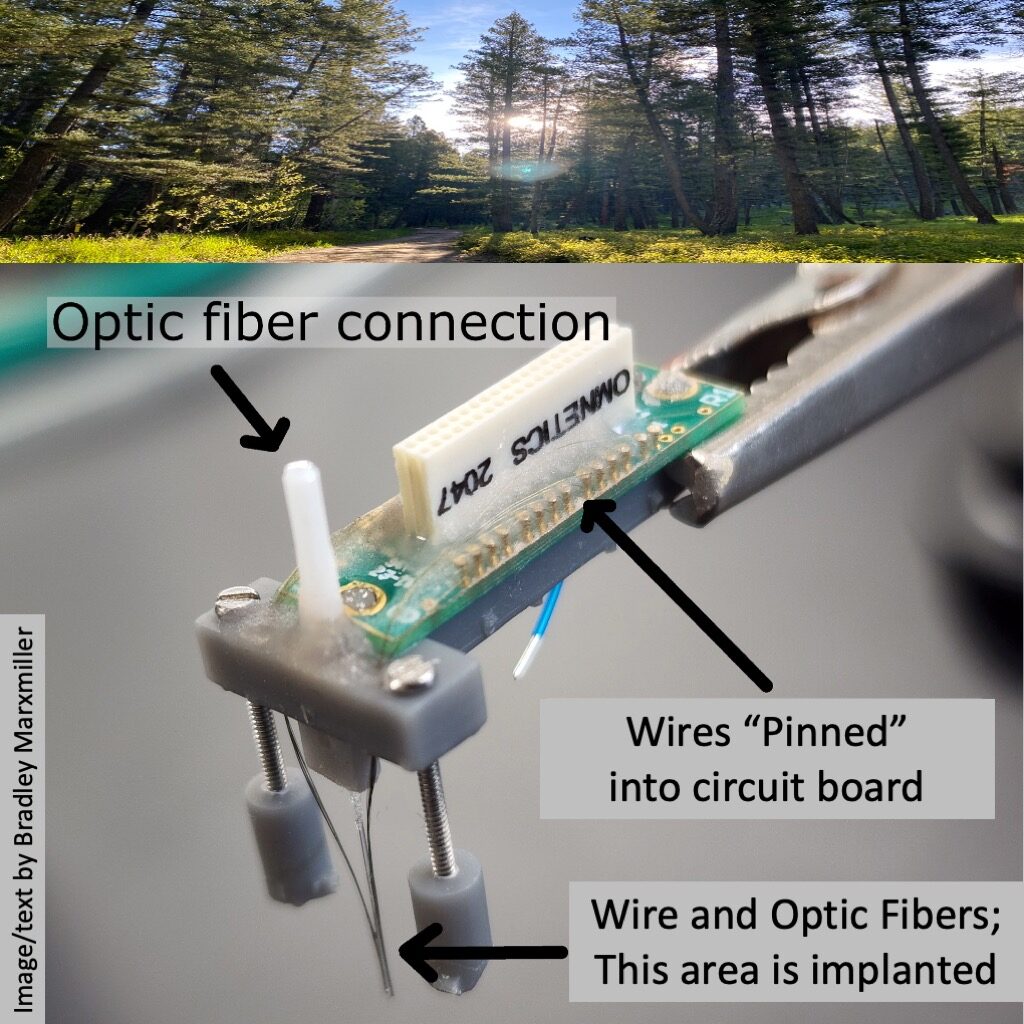
During the same procedure, we also install the headstage (the part of the apparatus attached to the skull) and the probe containing the optogenetic fibers and firing monitoring wires that are descended into the brain to collect data.
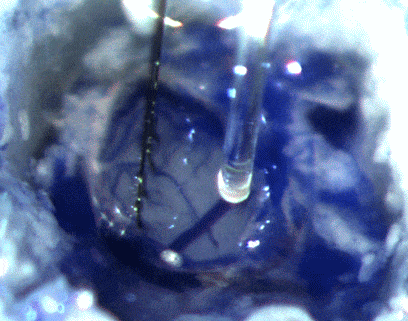
IMAGE SOURCE: Cambridge NeuroTech
LEARN MORE: Adeno-Associated Viral Vectors in Neuroscience Research
LEARN MORE: Adeno-Associated Virus (AAV) as a Vector for Gene Therapy
It takes a few weeks after the injection of the virus for the cells to become infected and express the new gates. Once these Arch gates are in, light (instead of neurotransmitters) will open ion gates, activating inhibitory VIP neurons and keeping other neurons from firing.
Once we have the mice expressing the Arch channels, the surgical site has healed, and we are getting the data from the implant wires, we can start the experiment. In general, we give the mice a task that they must complete to get a reward (water restriction cessation, sugar, ext) or a punishment (usually withholding the reward or a bright light flash in our lab) if the task is done improperly. We can then repeat the task with a laser that is set to the right wavelength to inhibit the VIP cells. By repeating this process over many days with many animals we are able to get a sizable portion of neurons to analyze.
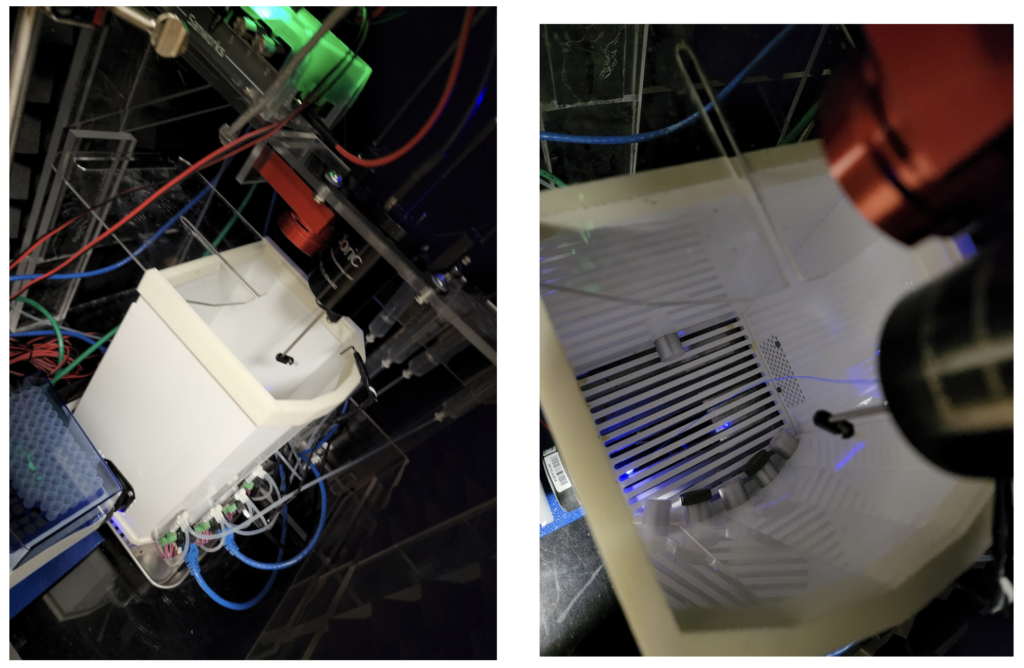
The mouse testing area, a “B pod” box with optogenetic wire included.
The differences between both behavior and the neural data we record potentially gives us insight, in the same way lesioning has: are those cells important for the task? If we inhibit firing, can we change the behavior of the mouse? Beyond behavioral changes, the wires that we implant pick up the electrical signals given off by neurons firing, showing up as a spike of electrical activity. These spikes can be aligned to stimuli and events, giving us further insight into what happens in the region or circuit connected to the VIP neurons when they are or aren’t active.
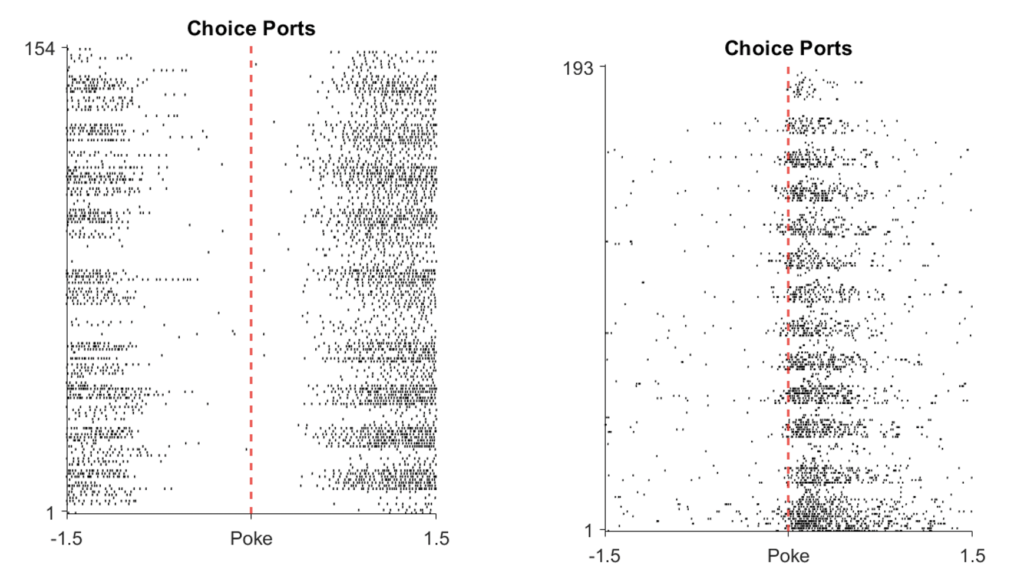
A neuron that does not fire when the mouse pokes something with its nose (left) vs one fires when a nose poke happens (right), made with Matlab by the post author.
Technology, such as optogenetic manipulation, is paving the way for current and future scientists to unlock the secrets of the brain.
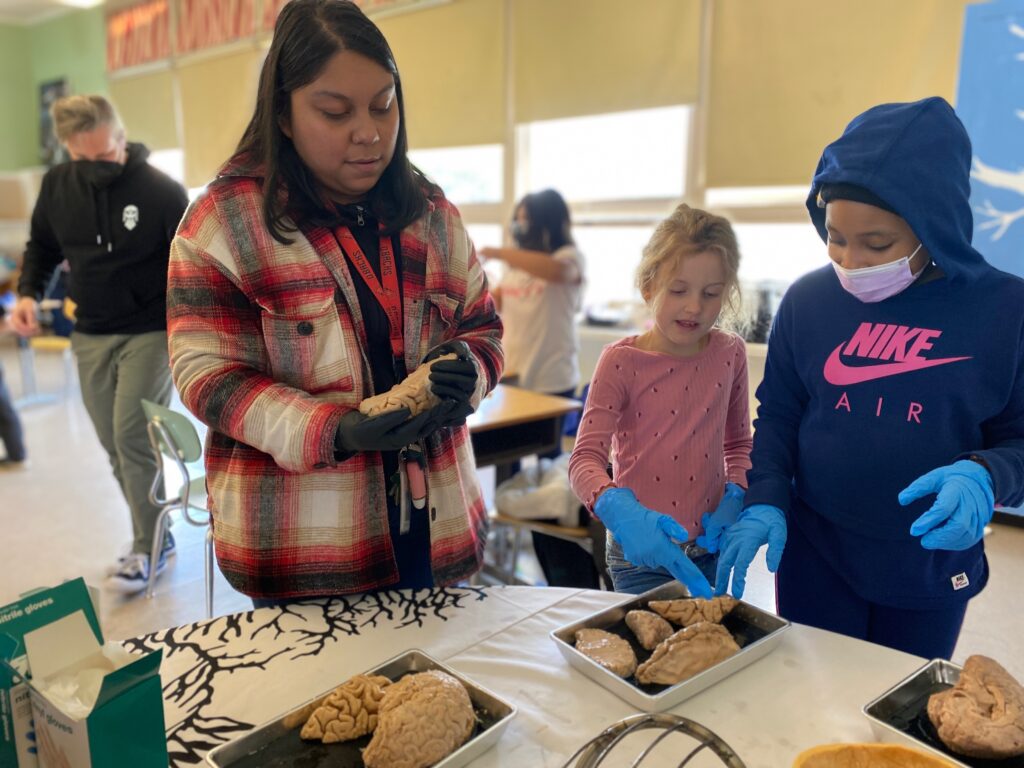
We’ve come a long way from learning about large areas of the brain through misfortune and mishap. While we can now target small circuits and specific cells for study, there is still an unimaginable amount of work to be done if we are going to answer: why do we think the way we do?
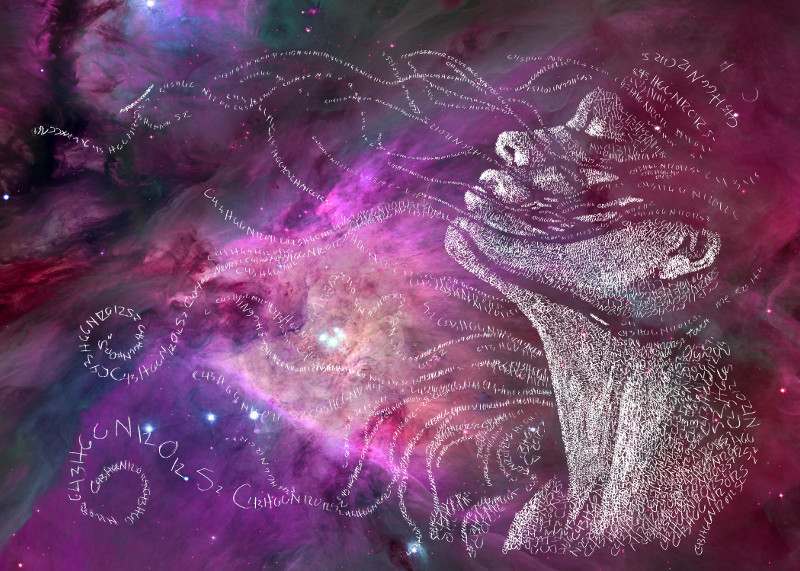
Touch, by Sienna Art Studios
Thank You
The good news is that science is collaborative by nature and anyone who has enough drive and desire can find a way to contribute. I’d like to thank Dr. Atheir Abbas and Dr. Alex Sonneborn for enabling me to do that, providing me with invaluable guidance on science and research skills. I’d also like to thank my funding source, NIH Build EXITO, for enabling this exciting opportunity to gain experience.
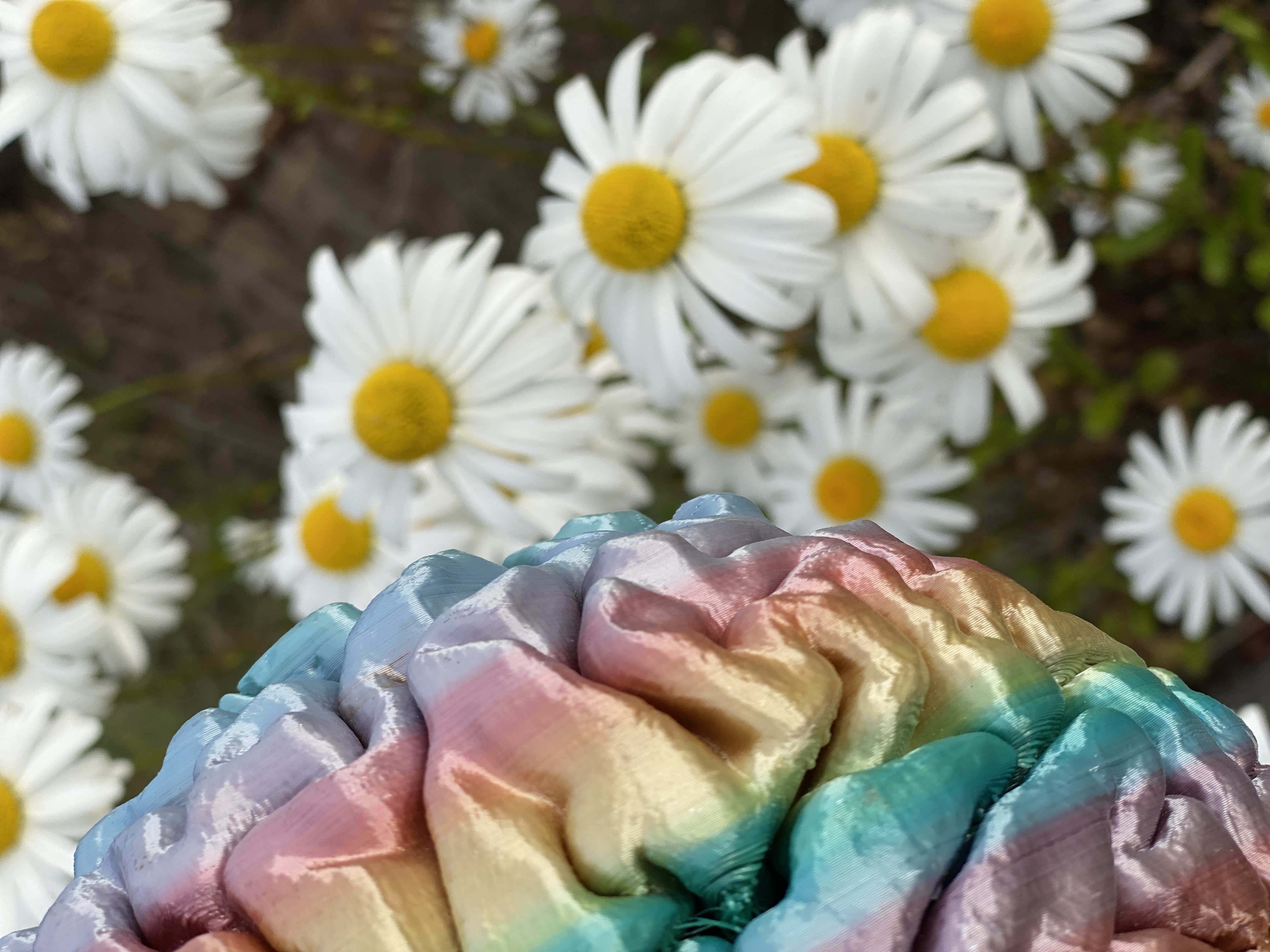