Post by Tiarra Jacoby, undergraduate at Portland State University
Volunteering at Legacy Research Institute (LRI) for Dr. Haiying Shen in the R.S. Dow Neurobiology Laboratories for the past few months has been an unforgettable experience. From day one, the research associates I’ve worked with have made me feel right at home in the lab, and I’ve gained more hands-on experience than I could have ever imagined for a short period of time.
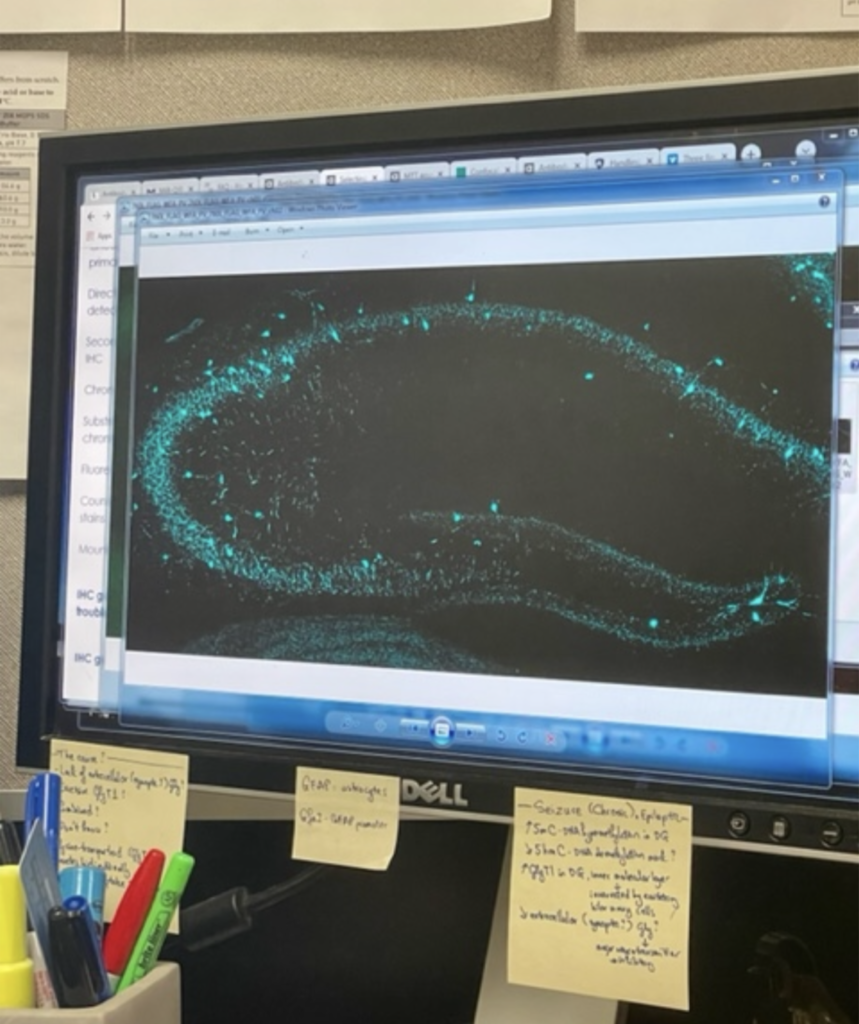
LEARN MORE: A Legacy of Links
The great thing about being surrounded by scientists is that any and all questions are always welcomed; the lab is an incredible learning environment for everyone! Before I was presented this opportunity, the idea of research felt exciting, but somewhat daunting. However, my experience at LRI has given me the tools and confidence to learn new, complex procedures and techniques involving immunohistochemistry, genotyping, and imaging analysis, that I can hopefully take with me into a research career.
Research on Temporal Lobe Epilepsy
Epilepsy and Glycine Transporter 1; a New Potential Target for Drug Development
Dr. Shen’s lab currently focuses on temporal lobe epilepsy (TLE), and how to prevent or reduce the development of seizures – a project he has been working on for several years. He is an expert in the field of adenosine and glycine-based mechanisms in neuropsychiatric disorders, and has published over 40 peer-reviewed papers!
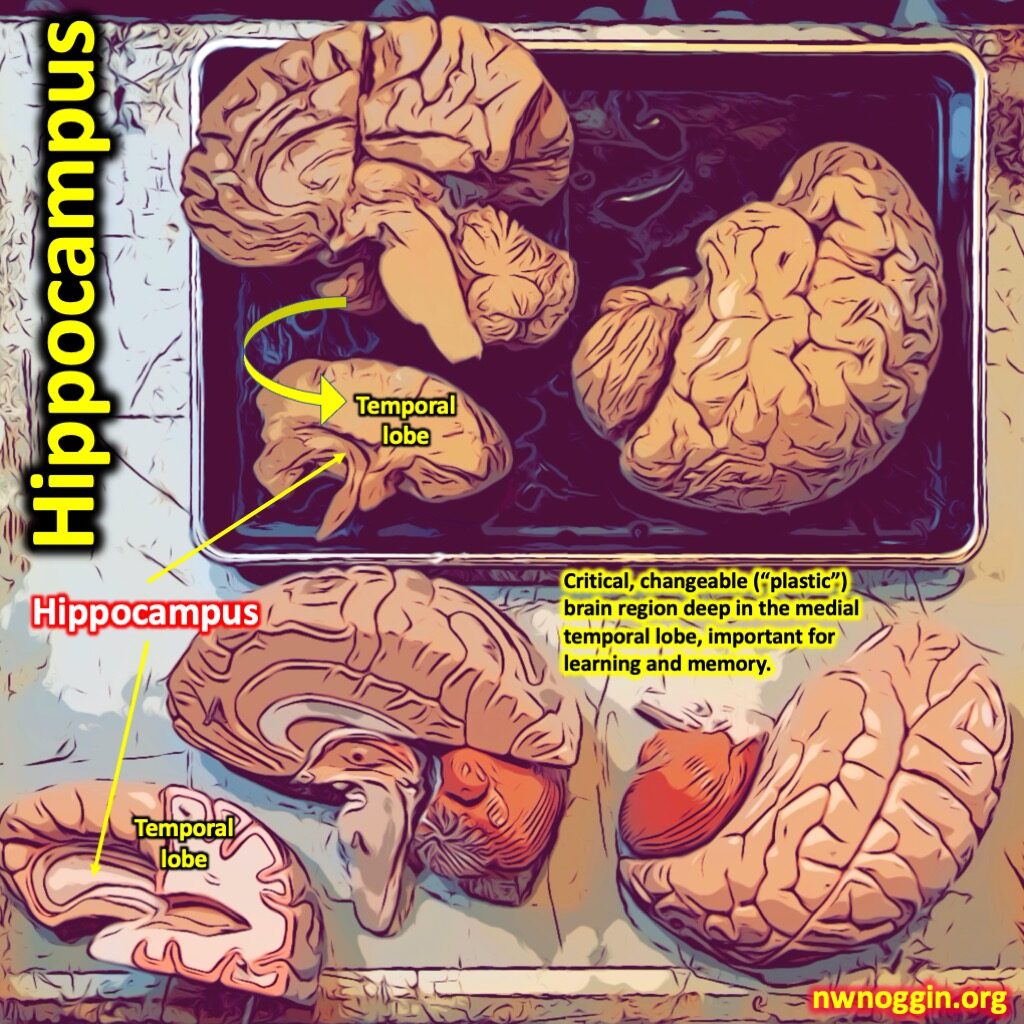
Over time, TLE can cause structural changes to the hippocampus, where many seizures begin, and can even cause sudden unexpected death in epilepsy (SUDEP). Chronic seizures increase the risk for developing cognitive symptoms that disrupt mood, memory, and daily life. Current drug treatments for epilepsy are hampered by major cognitive side effects as well. The goal of Dr. Shen’s research is to combat the detrimental effects of TLE by discovering a novel anticonvulsant treatment with pro-cognitive properties by looking at the issue from a new mechanistic angle: glycine transporter inhibitors.
The Role of Glycine
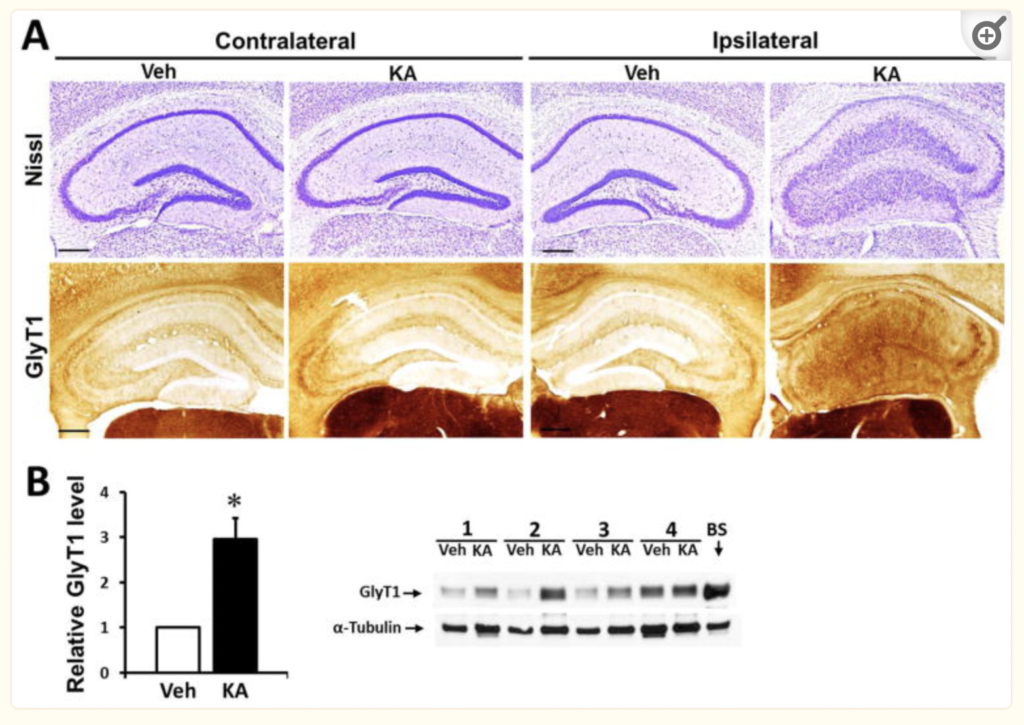
Glycine and its receptors in the hippocampus play an important role in regulating excitability in the central nervous system, while TLE is a disorder that disrupts the homeostasis of glycine and other neurotransmitters and neuromodulators. One study, authored by Shen and published in 2015 – which his current research is building upon – looked at the synaptic dysregulation of glycine within the hippocampus of epileptic brains as a new target area for drug treatment. In the hippocampal synaptic cleft, the availability of glycine is largely controlled by reuptake glycine transporter 1 (GlyT1, which is found in both neurons and astrocytes in the hippocampus). The hypothesis of this study was that an irregular expression of GlyT1 and the resulting disruption of synaptic glycine equilibrium is associated with epilepsy. In support of this claim, the genetic deletion of, or “gene knockout” (KO) of GlyT1 in the hippocampus showed an increased threshold for seizures in mice. Further, seizures were also suppressed by administration of a glycine inhibitor. The study concluded that GlyT1 overexpression constitutes a new target for therapeutic intervention, and that GlyT1 inhibitors may serve as a new, more suitable class of drugs for the treatment of epilepsy, similar to what has been in clinical development to treat symptoms of schizophrenia.
If you’d like to learn more about this research, please click the link!
LEARN MORE: Glycine Transporter 1 is a Target for the Treatment of Epilepsy (nih.gov)
Day to Day in the Lab
Each day spent in the lab is unique!
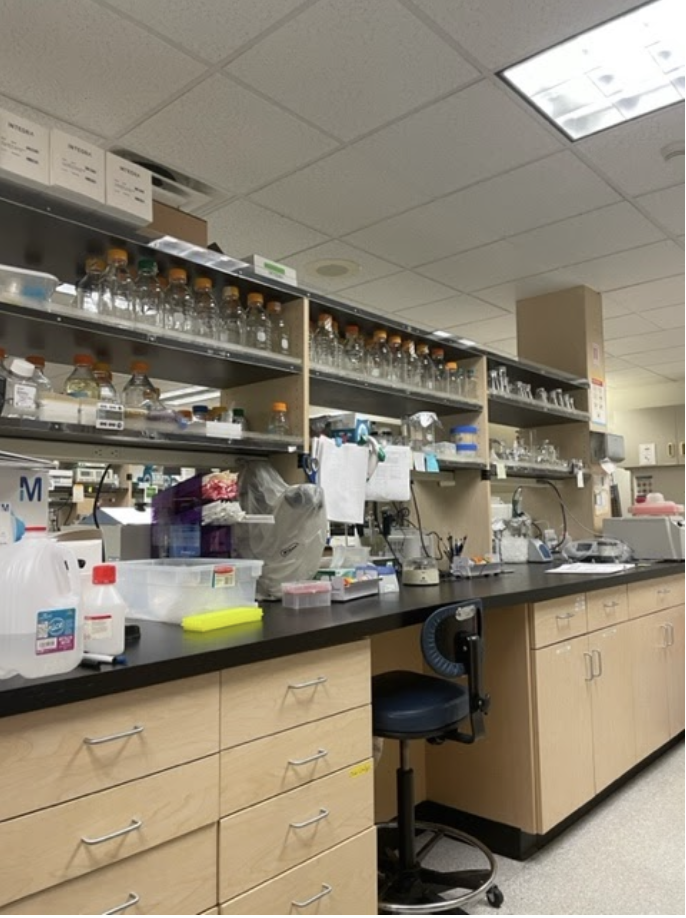
Now that you have a general understanding of the concept behind the research, I’d like to tell you more about the day-to-day that goes on behind the scenes, which leads to a paper like this being published. Although as a volunteer, I did not participate in handling live animals, I was able to observe, and work closely with animal tissues. One aspect of my research experience that I enjoyed was that each day is unique, with different tasks to complete. The overall breakdown of the project goal is to first ensure that we are working with GlyT1 knockout mice through genotyping. Then, introduce epilepsy via intrahippocampal injections to both KO and wild type (“WT”) mouse groups. Next, we monitor seizures via electroencephalogram (EEG), and assess GlyT1 activity via imaging. Finally, glycine inhibitors are introduced to determine their effect on chronic and acute seizures. Here’s what some of those processes look like.
Genotyping
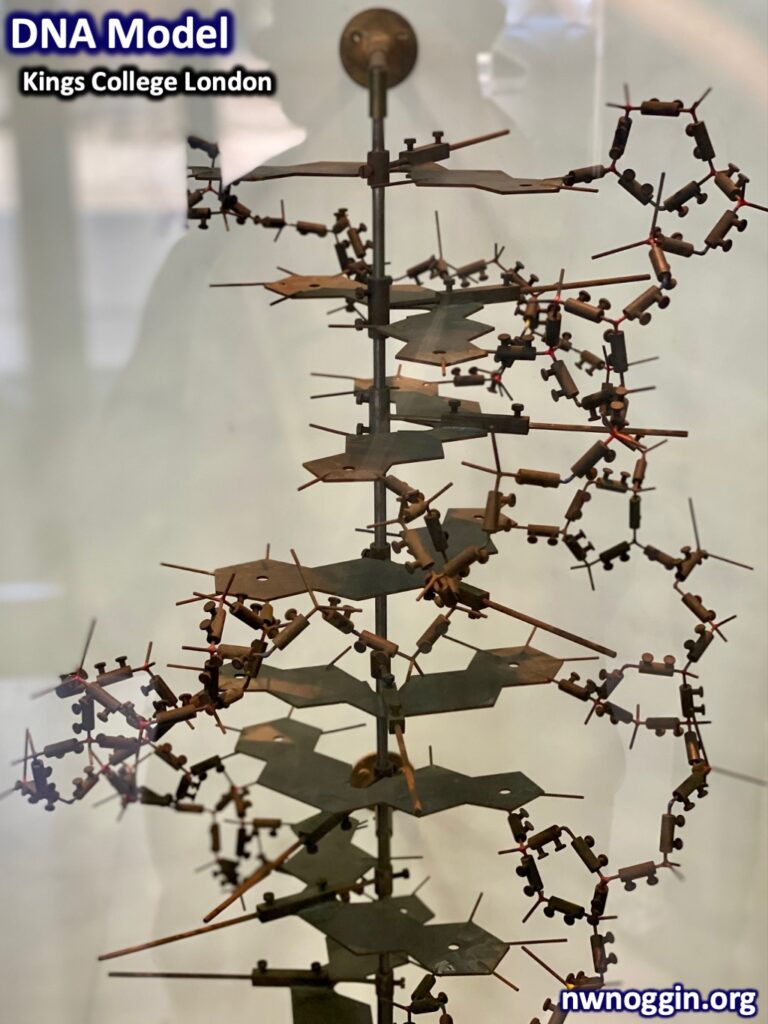
Genotyping is an important tool for this project, used to confirm that the mice we are working with are indeed genetically modified to have GlyT1 knocked out (“GlyT1-KO”), in order to observe this effect on suppressing seizures. The process involves first cutting a small portion of a mouse tail, and using a diluted lysate solution to dissolve tissues and break apart proteins for a PCR reaction. The PCR reaction uses specific primers to elongate a gene of interest – in this case Cre, which encodes Cre recombinase protein. We can determine that we have a GlyT1-KO mouse if its genotyping analysis comes back cre-positive. Here’s why: The Cre recombinase protein is able to cut out specific DNA sequences and catalyzes site-specific recombination between two flox sites in the DNA. Between these flox sites for our purposes are the genes that encode GlyT1, thus creating a GlyT1-KO. This is done for specific locations of the brain, for both neurons and astrocytes; the CamKII protein coding gene promoter is neuron-specific in the hippocampus, and EMX1 encodes proteins found in both neurons and astrocytes in the hippocampus. I should note that while WT mice are normally cre-negative, the mice we are working with come from a lineage of previously genetically altered, or KO mice, but we still need to confirm that they are cre-positive (in other words, lack GlyT1).
The PCR reaction prepares the proteins for gel electrophoresis, which helps us qualitatively visualize if Cre is present or not from our mice tail samples (Cre positive = GlyT1-KO). Gel electrophoresis works by separating mixtures of DNA, RNA, or in our case, cre recombinase proteins, as they are pushed through a porous gel by an electrical current, based on their molecular size. Western blot is another gel electrophoresis technique used for quantitative analysis, or how much of a protein is present.
Once the mice models are confirmed, both the GlyT1-KO mice and WT mice receive an intrahippocampal injection of kainic acid to induce temporal lobe epilepsy. The controls receive an intrahippocampal injection of saline. After chronic seizures are induced, the differences among the two epileptic groups (GlyT1-KO and WT) are compared, using EEG and imaging. Monitoring seizures via EEG shows adequate suppression of seizures in GlyT1-KO mice, as compared to WT epileptic mice, suggesting that GlyT1 inhibitors may be an effective new treatment for epilepsy!
Collecting tissue for analysis
Slicing brains
The next step is to collect tissue samples from these brains to directly demonstrate GlyT1 expression from both groups through microscopic imaging. On one of my first days in the lab, I learned how to slice mice brains, which would later be stained and mounted onto slides for imaging. First, the occipital lobe is sliced off to provide a flat surface for the semi-frozen brain to be mounted, using a glue-like solution called OCT (optimal cutting temperature).
The slicing machine, called a cryostat, is practically an intricate mini-deli slicer, producing extremely thin (30 nm thick) slices for each crank of the lever attached.
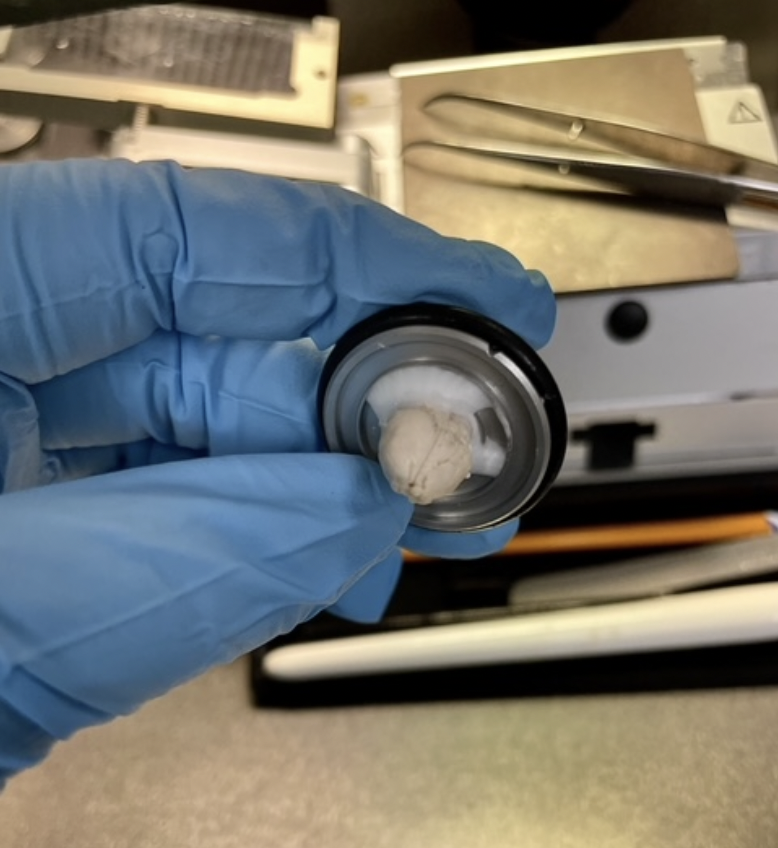
As you’re slicing to get to the area of the brain of focus (the hippocampus in my case), you must make careful adjustments so that you get a symmetrical image.
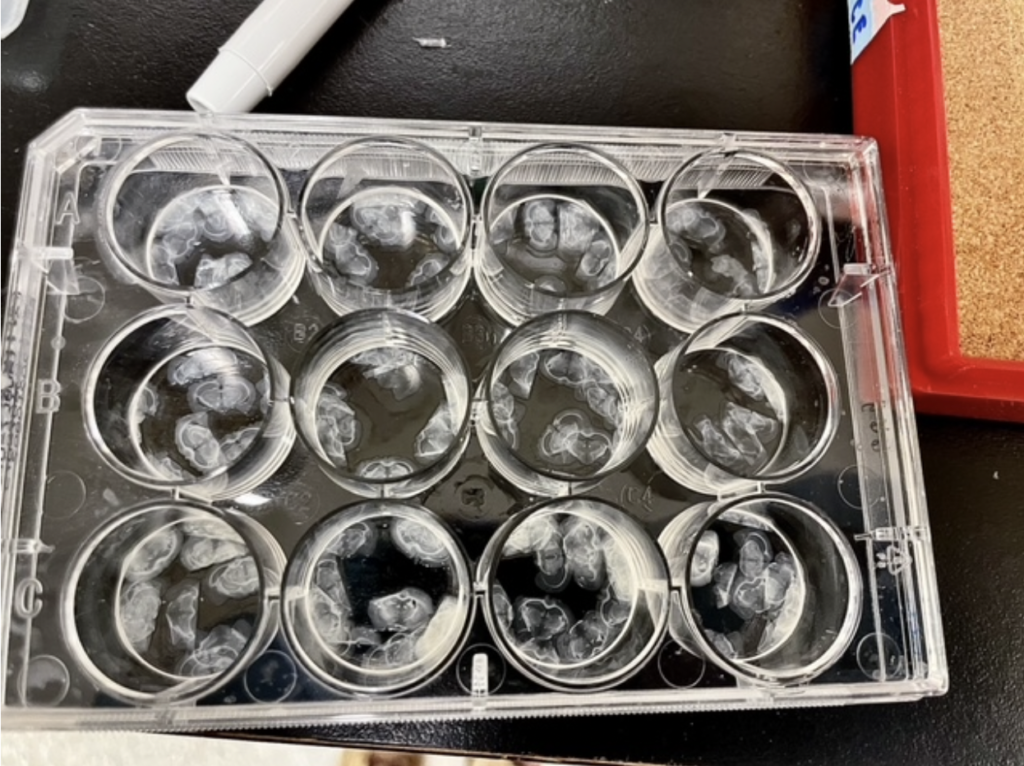
Once you’ve reached the brain area of interest, it is time to start collecting slides.
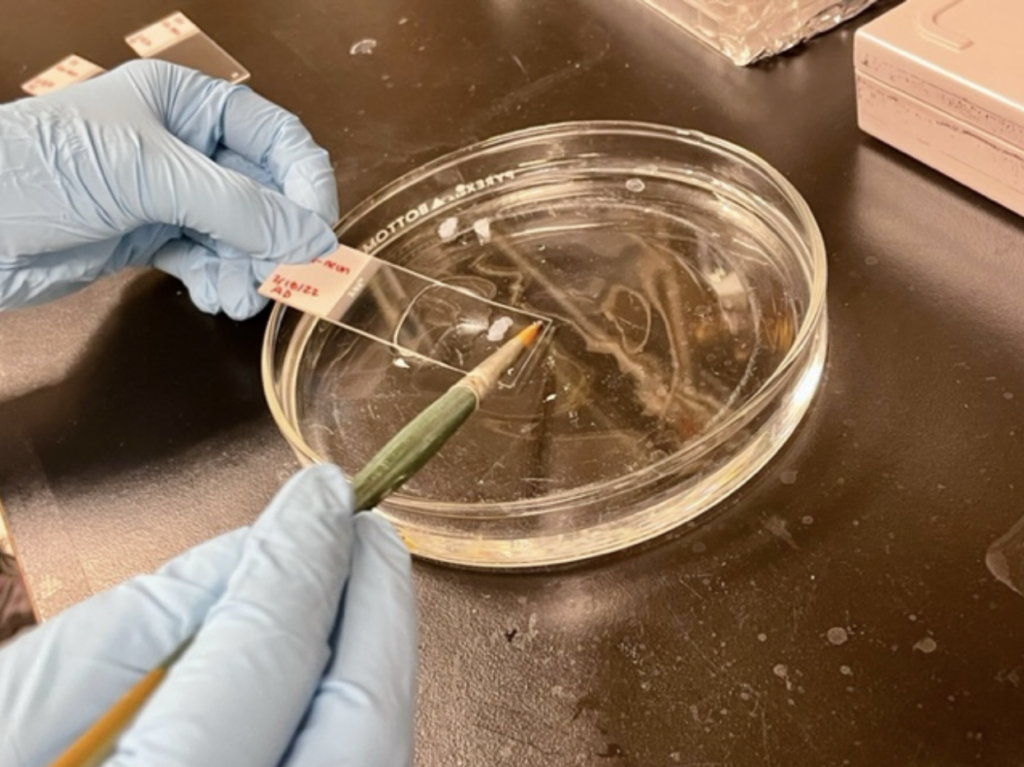
This is done with a small paintbrush to pick up the delicate slices, which are then placed in solution (phosphate buffer solution or PBS) for preservation. Many slides within the range of interest are collected in case any become damaged; like I said, they are tiny and fragile, but hold so much information!
Immunohistochemistry
The last step before imaging is immunohistochemistry (IHC), a type of immunofluorescent staining. IHC utilizes antibodies, which bind to antigens, in order to visualize biological tissues, such as GlyT1, through a microscope. The antibodies used are sourced from various different animals such as rats, rabbits, goats, etc. and must be sourced from a different animal than the antigen, so that it binds – like how the antibodies our bodies make react with foreign antigens.
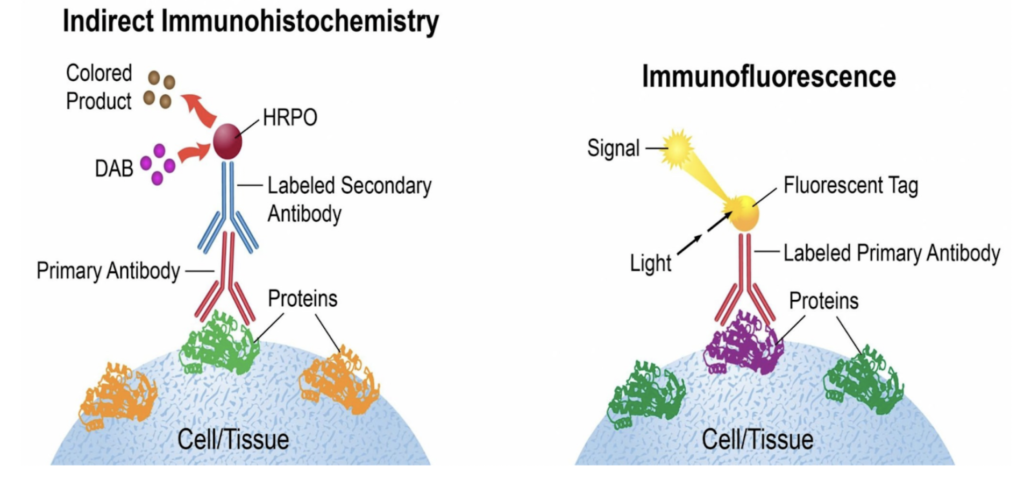
Here’s a link if you’d like to learn more about IHC: Antibody basics training | Abcam (myabcam.com)
This is a multi-step process.
Day one of IHC consists mostly of washing the tissues, and using a blocking buffer that reduces background interference, so that the antibody binding is clearly visible. After incubation, day two of IHC consists of adding a secondary antibody and staining to ensure that the images will highlight the target we’re looking for (GlyT1). For our case, we use NeuN, which stains neurons, and GFAP, which stains astrocytes. The antibodies used show up on microscopic imaging at specific wavelengths of light so that we can observe GlyT1 in neurons at a certain wavelength, and GlyT1 in astrocytes at another wavelength. So, in theory, images of mice brains with genes (EMX1 and CamKII) that are Cre +, (meaning GlyT1 was knocked out), will show up with less visible GlyT1 in neurons and astrocytes of the hippocampus than in WT mice with the genotype Cre -. With GlyT1 knocked out, that irregular expression of glycine in the synaptic cleft of epileptic brains is returned to homeostasis, thus helping to control chronic seizures. Later in the study, GlyT1 inhibitor treatments will show the same effects without having to knock out any genes.
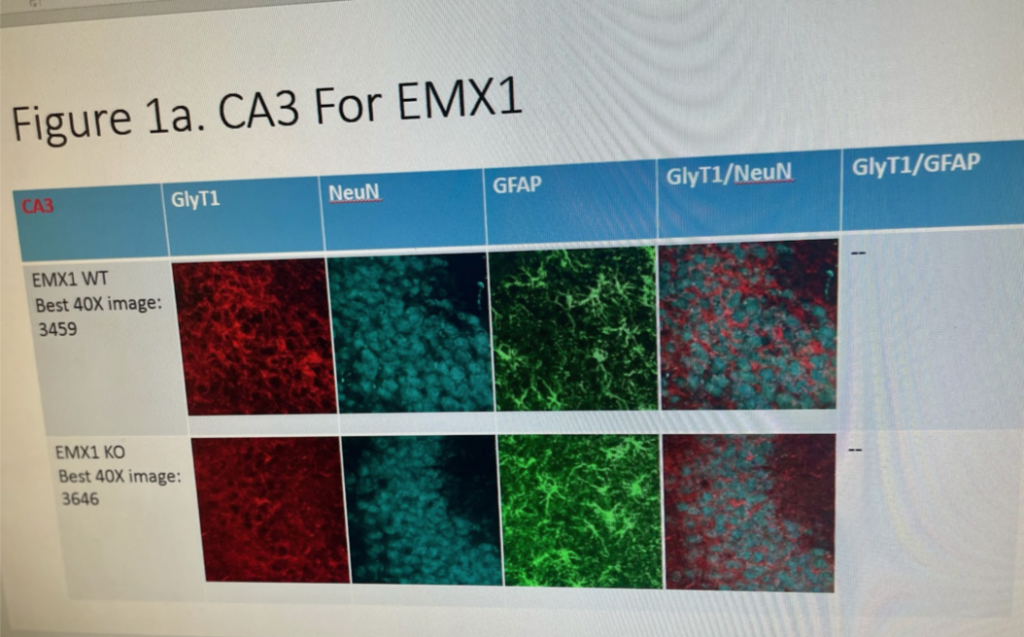
Once the brain slices are stained and carefully mounted onto slides, a coverslip is applied using nail polish along the sides for adhesion.
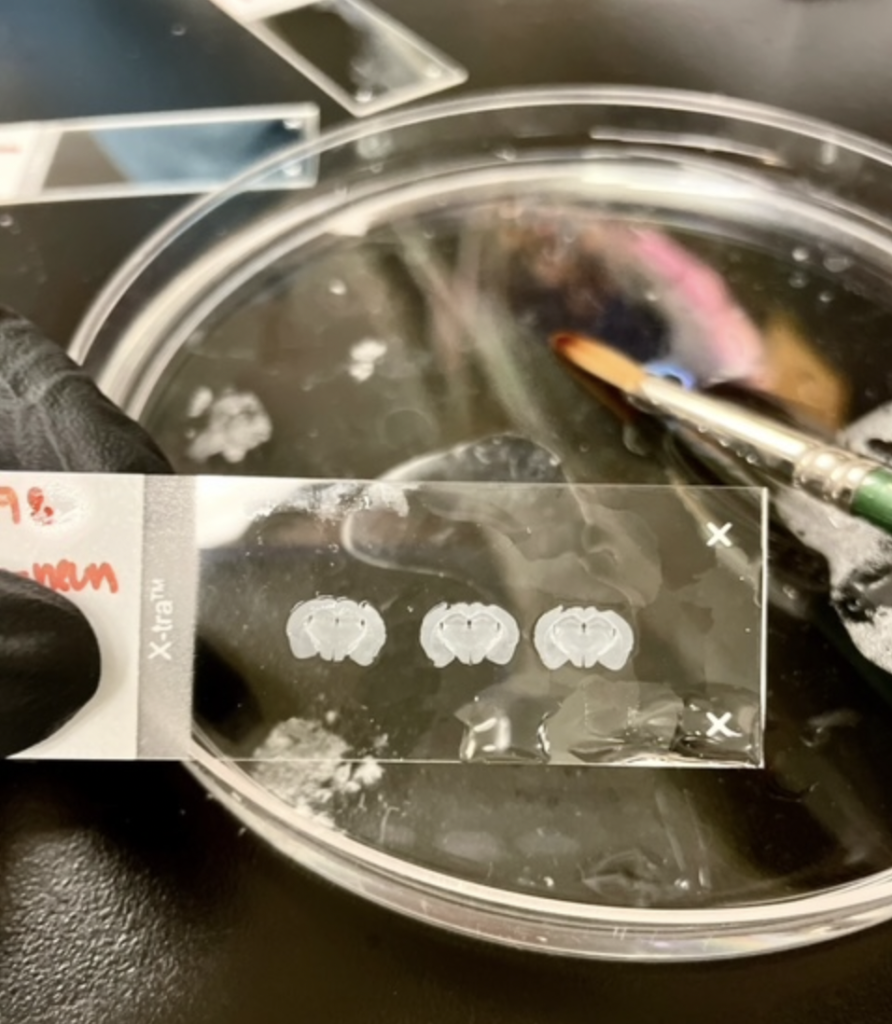
Then, an inverted confocal microscope, which uses lasers and specific wavelengths of light related to the different types of staining used in IHC, is used to create beautiful images of the brain.
If all procedures leading up to this point were done correctly, imaging is where all of the hard work comes together!
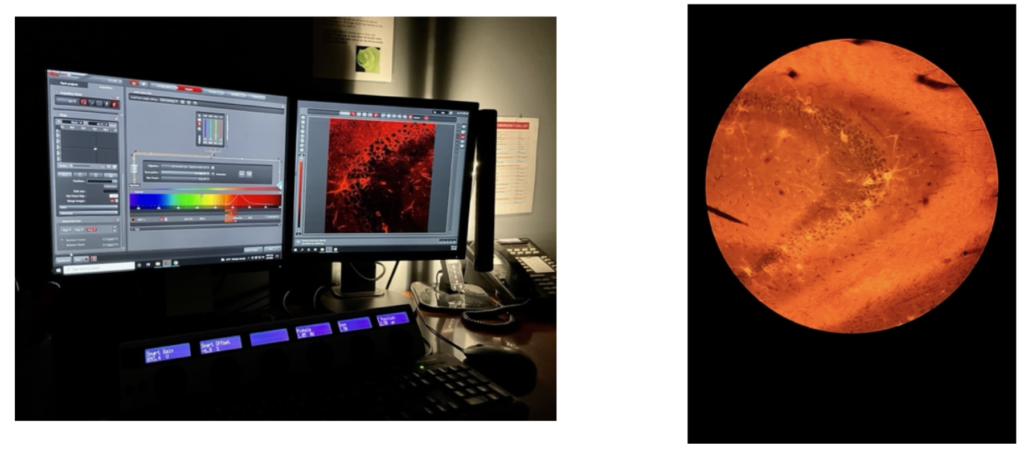
Not only does imaging show us the results we’re looking for, but it also tells us a lot about the quality with which all of our procedures leading up to this point were done with. Once we have images of the hippocampal GlyT1’s in both astrocytes and neurons for WT and GLYT1-KO mice, we can compare this data to the EEG results from the same mice groups to demonstrate the role that glycine has in TLE. Because GlyT1-KO mice show a great reduction in seizures, and imaging from these mice presents a reduction in GlyT1 expression, glycine inhibitors were also shown to be a suitable strategy to controlling chronic seizures associated with TLE.
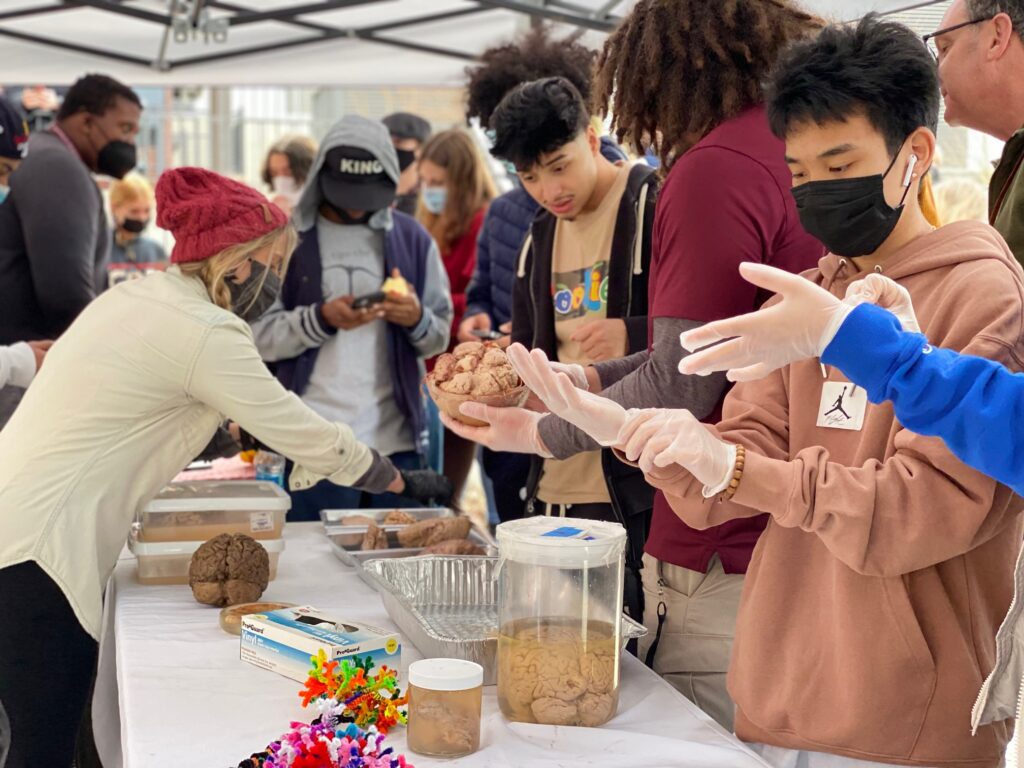
Thank you!
It has been a great pleasure to experience and learn so much during my time at LRI, which I plan to continue. I would like to give a big thank you to Dr. Bill Griesar, who helped me discover this opportunity, Dr. Haiying Shen for onboarding me to gain research experience, and each of the research associates who helped me along the way. If you are interested in research, a great way to get started is to explore research institute websites, find a scientist or project that interests you, and start reaching out to see how you can get involved. In my experience, many people are happy to help enthusiastic students by at least pointing you in the right direction. NW Noggin is another wonderful resource for establishing connections that may help you begin your research journey!